How the Proton Got its Spin
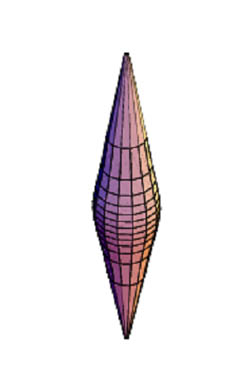
Inside protons are quarks. Experiments can’t reveal them directly, but somehow the behavior of these internal constituents generates a proton’s properties. Making that connection, however, is no easy matter. A theoretical paper in the 8 August PRL illuminates the proton’s interior by turning an esoteric mathematical description of quarks into a visual form. The pictures provide tangible detail on how internal dynamics contribute to the proton’s spin.
When the quark picture first emerged in the 1960s, physicists assumed that the three quarks inside a proton each move in a spherically symmetric fashion, creating an object that in many respects acts like a little ball. According to this view, the observed spin of the proton arises simply from the intrinsic spins of the quarks. In the late 1980s, however, experimental evidence began to show that much of a proton’s spin comes from so-called orbital motion of the quarks relative to each other, rather than from their individual spins. In addition, it became apparent that quark-antiquark pairs and other particles continually flit in and out of existence inside a proton, all influencing the proton’s characteristics.
In 1996, Xiangdong Ji of the University of Maryland in College Park introduced a mathematical tool that he called the Generalized Parton Distribution (GPD) and used it to relate experimental data to the configuration of particles inside a proton. But the GPD, he says, is “abstract [and] hard to understand” in an intuitive way. As a tool to explore the origin of proton spin, he adds, it “seemed to be far more complicated than necessary.”
To understand Ji’s new way of depicting the internal structure of a proton, recall the behavior of quantum particles. An electron in a hydrogen atom, for example, is often represented by an “electron cloud” image. The dumbbell-shaped “p-orbitals” of a hydrogen atom show where the electron is most likely to be if the atom is prepared in the quantum state called p. Similarly, quarks within a proton are quantum particles occupying some volume of space rather than a specific location. Because of the uncertainty principle, a quark’s momentum is likewise “fuzzy.”
Ji’s latest trick turns his mathematical GPDs into images that look something like orbital diagrams for a hydrogen atom. He calls them “color filters” because they pick out only the quark motion at a certain momentum, just as a filter in photography shows the scene at a certain color of light. To understand this filtering, think of cars moving in or out of a city during rush hour, Ji suggests. If you look at all cars together, you will see a roughly uniform pattern, but if you image only those vehicles moving north at 30 miles per hour, say, a handful of distinct streets will stand out.
Each of Ji’s filter images gives a picture of the most likely locations of quarks if one only observes at a specific value of momentum. The pictures show that quarks with some particular momentum do not necessarily occupy a spherically symmetric region. Adding up the pictures from all quark momenta does give an exactly spherical distribution. But the non-spherical shapes of some of the pictures show that quark motion is not entirely random–it depends on location. In general, says Ji, such a connection between quark momentum and position implies an overall rotation of the quarks around one another, which we observe as proton spin.
Ji’s latest work is “a real step forward,” says Tim Londergan of the Indiana University in Bloomington. By giving a direct idea of quark location, he says, Ji’s methods can give physical meaning to mathematical concepts that are not easy to interpret.
–David Lindley
David Lindley is a freelance science writer in Alexandria, Virginia.