Landmarks—Forgotten Black Hole Birth
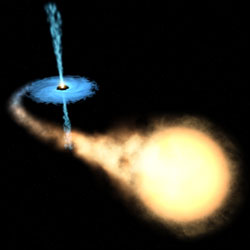
APS has put the entirePhysical Review archive online, back to 1893. Focus Landmarks feature important papers from the archive.
Had J. Robert Oppenheimer not led the US effort to build the atomic bomb, he might still have been remembered for figuring out how a black hole could form. His 1939 Physical Review paper, written with graduate student Hartland Snyder, described how a star might collapse into an object so dense that not even light could escape its gravitational clutches. The paper was hardly noticed until the 1960s, when astrophysicists began to seriously consider that such extreme objects might exist. John Wheeler of Princeton University then came up with the name “black holes” for these now standard elements of astrophysics.
In the 1930s, as astronomers began to ponder the future of a star whose nuclear fuel had run out, they ran into difficulty describing its fate. When energy generation within a star falters, its own gravity begins to take over, compressing the star’s mass. Our own sun will end up as a white dwarf, whose core is a dense mix of atomic nuclei in a sea of electrons. But the fates of larger stars were less clear.
Researchers understood that a white dwarf with too much mass isn’t stable, and its own immense gravity should compress it into something denser, possibly made entirely of neutrons. In a paper published early in 1939 [1] Oppenheimer and George Volkoff, both of the University of California at Berkeley, proved that neutron cores, like white dwarfs, could not be indefinitely heavy. In this paper they combined general relativity–Einstein’s theory of gravity–with a quantum mechanical description of a pure neutron fluid. But they could find no stable solution to the equations if the neutron core had more than about 70% of the sun’s mass. Astrophysicists now know such large cores result from the deaths of stars several times as massive as the sun. For a larger core, collapse must proceed further. But into what?
Oppenheimer and Snyder answered that question in a paper later in 1939. Since static solutions to their equations seemed to be impossible, they looked for solutions where the structure of the object never stopped changing in time, representing continued contraction. Theoretical physicists at the time had little experience applying Einstein’s equations under such extreme conditions–not to mention a total lack of mathematical computer power–so the team had to make many approximations and educated guesses along the way. Still, their brief paper arrives at essentially correct conclusions about the formation of what we now call a black hole.
The matter in an extreme collapsing object, Oppenheimer and Snyder say, would retreat inexorably inward. Meanwhile, any escaping radiation would suffer an increasing gravitational redshift, drifting to ever longer wavelengths as it fought an uphill battle against gravity. An outside observer would see the collapsing object become redder and fainter. “The star thus tends to close itself off from any communication with a distant observer; only its gravitational field persists,” the authors conclude.
“It fascinates me why that paper was neglected for so long,” says Saul Teukolsky of Cornell University in Ithaca, New York. There were some loose ends, he explains. It seemed possible that some extraordinary source of pressure might prevent contraction all the way to a black hole, but Wheeler and others, reviving the subject in the 1960s, showed that there was no way out. Teukolsky says that among many astronomers and physicists there was an instinctual revulsion against unlimited collapse, and that Lev Landau of Moscow University even suggested modifying quantum mechanics to make sure it couldn’t happen. The Oppenheimer-Snyder paper “goes against the prevailing thought of the time,” he says. “It really stands out.”
–David Lindley
David Lindley is a freelance science writer in Alexandria, Virginia.
References
- J. R. Oppenheimer and G. M. Volkoff, “On Massive Neutron Cores,” Phys. Rev. 55, 374 (1939)