Nobel Focus: Limited Freedom for Quarks
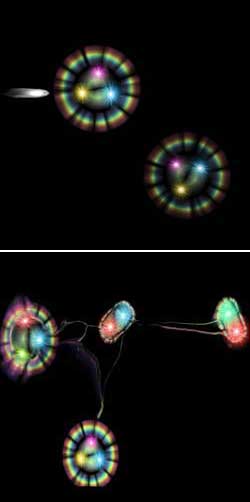
This year’s Nobel Prize in Physics honors three theorists whose insight resolved what had appeared to be an intractable subatomic paradox. By the late 1960s physicists believed that protons, neutrons, and many other particles were made of quarks that remained firmly locked together and never appeared in isolation. Yet experiments indicated that quarks within protons were only loosely bound together. In 1973, David Gross, now at the University of California, Santa Barbara; Frank Wilczek, of the Massachusetts Institute of Technology in Cambridge; and David Politzer, of the California Institute of Technology in Pasadena, published papers in PRL and Physical Review D that explained how a single force acting between quarks could account for these seemingly contradictory facts.
Researchers then had no good theory of the so-called strong nuclear force that holds quarks together. To probe the mechanism of quark confinement, experimenters blasted protons with high-energy electrons. The more energetic was the electron beam, the more the electrons reacted as if they were encountering particles moving freely within the proton, hardly stuck together at all. Physicists coined the term “asymptotic freedom” to describe the way quarks seemed to interact more feebly at higher energies. This phenomenon was surprising because the highest-energy collisions probe the strong force at the shortest distances, where researchers expected to encounter its most robust effects–just as two electrically charged particles feel a greater force the closer they are to each other.
The apparent contradiction between asymptotic freedom and quark confinement created “chaos and turmoil” in the theory of the strong interaction, recalls Chris Quigg of the Fermi National Accelerator Laboratory in Batavia, Illinois. David Gross, then at Princeton University, and his student Frank Wilczek set out to satisfy themselves that no legitimate quantum theory of particle interactions could display asymptotic freedom. To universal surprise, they found exactly the opposite, discovering a class of theories in which the strength of the interaction becomes steadily smaller at shorter distances. Like a ping-pong ball tethered by elastic to a wooden paddle, the farther you try to separate a quark from its neighbors, the stronger the attraction becomes. David Politzer, then a graduate student at Harvard University, independently came to the same conclusion, and their papers appeared back-to-back in PRL.
Within months, a quantitative strong interaction theory followed. In Physical Review D Gross and Wilczek showed that the strong force acted on a property called color, helping to flesh out a theory of “quantum chromodynamics” previously postulated by Murray Gell-Mann, then of the California Institute of Technology.
In this scheme, quark-antiquark pairs form kaons, pions, and other mesons, while triplets of quarks form protons, neutrons, and numerous heavier cousins that appeared in accelerator experiments. Gross and Wilczek’s theory also hints at an explanation of why no isolated quark can be liberated: If you hit a proton hard enough, the energy simply creates a new quark-antiquark pair–a meson–rather than knocking out a free quark. Most physicists find this argument convincing, though there is still no complete theoretical proof for absolute quark confinement.
Armed with a calculable theory of strong interactions, physicists could begin to tackle questions that had seemed beyond their grasp, says Quigg. The proton’s mass, for example, arises because of the energy tied up in the strong binding of three quarks. Gross, Wilczek, and Politzer may not have found what they originally thought they would find, Quigg adds, but they got something “infinitely more wonderful.”
–David Lindley
David Lindley is a freelance science writer in Alexandria, Virginia.