Bake, Shake, and Shrink
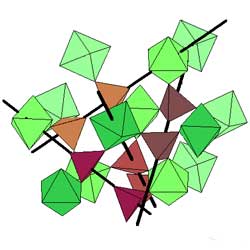
Almost every material swells when you heat it, but a handful of maverick materials do just the opposite, shrinking as they get hot. A team reporting in the 26 November PRL argues that complex vibrations in one unusual crystal structure conspire to produce the most striking known example of this so-called negative thermal expansion (NTE). The lessons learned may eventually lead to materials that remain the same size at all temperatures by combining elements with both positive and negative thermal expansion.
In most cases of NTE, the effect occurs over a limited temperature range, beyond which the material rearranges its atomic structure. Zirconium tungstate ( ) is unique because it exhibits NTE from close to absolute zero up to nearly 1000 degrees Kelvin while retaining the same atomic structure. The atoms in the crystal are arranged in a network of octahedra and tetrahedra. The overall structure is very open, with many possible modes of vibration, because the polyhedra connect only at their vertices, where they share atoms, and because each tetrahedron has one free “unshared” vertex.
In some materials with structures made of linked polyhedra, NTE occurs because the polyhedra can pivot at their shared vertices into a structure that fills space more compactly without changing any bond lengths. As the material heats, the polyhedra rock back and forth more violently between the more compact and less compact structures. If the material spends more time in the contracted state, it shrinks. But this motion stresses atomic bonds, so that with enough heat, NTE leads to a gross rearrangement of the structure in most cases.
Researchers had suggested that the free vertex of the tetrahedra in zirconium tungstate allowed volume-shrinking oscillations to occur much more easily than in other materials, without destroying the crystal’s structure, explains Zack Schlesinger of the University of California at Santa Cruz. Experiments pointed to a twisting motion of the tetrahedra. However, recent evidence indicated that the tetrahedra might also vibrate in pairs, moving toward and away from each other. Both kinds of motion seemed to be important in zirconium tungstate, but how they produced this extreme case of NTE was unclear, says Schlesinger.
To probe the full range of the material’s structural oscillations, Schlesinger and his colleagues aimed infrared light at a sample of zirconium tungstate and measured the spectrum of reflections, noting the frequencies where lattice vibrations absorbed some of the light. The team was looking for more modest information but was surprised to find a complicated set of infrared absorption lines well below the frequencies corresponding to stretching and bending of single bonds. Modeling the lattice in ball-and-spring fashion to deduce the cause of these new lines, the team concluded that the tetrahedra execute both kinds of motion: when they do the twist, they also pull their partners to and fro.
This work is “a significant step forward,” says Art Sleight of Oregon State University in Corvallis, because it shows how the two previously suggested vibrations combine to cause contraction. Schlesinger hopes that better understanding will lead ultimately to the ability to design new materials with NTE. Combining such a substance with conventional materials could, for example, lead to materials that hold microcircuits and remain fixed in size over a wide temperature range.
–David Lindley
David Lindley is a freelance science writer in Alexandria, Virginia.