Charged-Up Ice Crystals
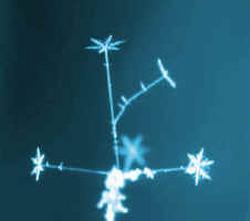
Snowflakes and many other crystals normally grow in fingerlike, “dendritic” patterns that physicists do not yet fully understand. In the 6 July PRL a team reports a new type of hypercrystallization process caused by electric fields: they describe ice “needles” that can grow at more than 10 times the normal rate. They show that a simple extension of crystallization theory explains many features of the accelerated growth and suggest the new method could be useful for controlling crystal growth in materials applications.
The intricate structures of snowflakes come from the natural growth patterns of crystals from vapor or liquid: branches grow upon branches–a treelike pattern known as “dendritic growth.” Although dendrites often appear in rocks and solidifying metals, as well as snowflakes, it was only a decade ago that physicists formulated a consistent mathematical theory for the simplest dendritic growth, says Kenneth Libbrecht, of the California Institute of Technology. “Crystal growth is a ubiquitous phenomenon, but it’s poorly understood mathematically,” he says.
To test and extend the current theory, Libbrecht and his Caltech colleague Victoria Tanusheva applied an electric potential to growing ice crystals and found that it accelerated the normal dendritic growth somewhat. But when they turned the voltage up above a certain value, the crystal growth suddenly sped up dramatically, to between 20 and 70 µm/s–more than ten times the normal rate–and the branching dendrites suddenly began growing as sharp needles.
When he applies a high voltage to an ice crystal, says Libbrecht, a strong electric field emanates from each advancing tip. The field is strong enough to “polarize,” or align, the randomly floating water vapor molecules, and the small tip size creates large field gradients that attract them. The effect pulls water molecules onto the crystal at a higher rate than normal, and Libbrecht and Tanusheva describe a mathematical extension of the conventional theory that accounts for this effect. But above a threshold voltage the theory breaks down–exactly at the point where they observe runaway crystal growth. At that point, say the authors, the electric field effects overwhelm the tip’s surface tension, which normally keeps the crystal growth in check. Old “cloud chamber” particle detectors used a related effect: a muon speeding through a supersaturated vapor blazes a trail of ions which cause liquid droplets to form along the particle’s path. The droplets can form only because each ion provides a sufficient electric field.
Kenneth Kliewer, of the Oak Ridge National Laboratory in Tennessee, calls the work “totally intriguing” because it demonstrates that external forces can dramatically affect crystal growth and because it leads to many more questions. Libbrecht and Tanusheva suggest that since their method allows precise control of the crystal growth rate, it may be useful for producing or studying a wide range of materials. They have also used the ice needles as a base for growing artificial snowflakes–“nobody’s ever made them in a well-controlled fashion in the lab,” says Libbrecht.