A Molecule of Light
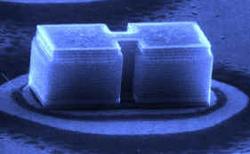
A micrometer-sized piece of semiconductor can trap photons inside it in such a way that they act like electrons in an atom. Now the 21 September PRL describes a way to link two of these “photonic atoms” together. The result of such a close relationship is a “photonic molecule,” whose optical modes bear a strong resemblance to the electronic states of a diatomic molecule like hydrogen. Photonic systems can be controlled and manipulated in ways that are not possible with regular atoms and molecules, so they have a wide range of applications–from probing the basic physics of molecular bonding, to building more efficient semiconductor lasers.
For many years physicists have probed confined electrons in nanometer-sized semiconductor regions called “quantum dots.” In contrast, the first photonic atom was fabricated just last year [1] by a collaboration led by Alfred Forchel, of the University of Würzburg in Germany, and Thomas Reinecke, of the Naval Research Laboratory in Washington, DC. But photonic microcavity structures might soon become as important as their electronic counterparts because they are easier to understand and easier to manipulate. The theoretical description of connected photonic atoms is not confounded by many-particle effects, such as the complicated electron-electron interactions in coupled quantum dots.
To make a photonic molecule, the group constructed two blocks of gallium arsenide, approximately 3 × 3 × 1 µm in size, to act as light-confining resonators. The blocks were linked together with a narrow bridge that allowed them to interact. A tiny quantum well in the center of each block produced light in response to laser pulses. The size and shape of the whole structure tended to enhance specific frequencies (optical modes) of this light as it reflected within the structure–essentially the same effect that causes electrons confined in atoms and molecules to assume a set of discrete energy levels. A fraction of the light escaped, which allowed the team to measure the intensity of the photonic molecule’s light over a range of wavelengths. They observed peaks in the spectrum that corresponded to the optical modes.
As they made the bridge between the two photonic atoms shorter, the lowest frequency mode split into two. Reinecke and his colleagues suspected that the splitting was due to the photonic atom states transforming into photonic molecule states, just as the energy levels of two hydrogen atoms split when they bind to become a molecule. In a hydrogen molecule, the two energy states are called “bonding” and “antibonding” orbitals, and they have distinct shapes. To test the analogy, the team carried out numerical calculations of the electric field distribution inside the photonic molecule and found patterns that were similar to the bonding and antibonding orbitals in a hydrogen molecule. The numerical calculations also agreed with their experimental determination of the electric field patterns in the cavity. These patterns could be deduced by measuring the intensity of light emitted at different angles.
This simple photonic molecule is just the first step toward fabricating even more complicated structures, atom by atom, in a way that is impossible to do with electronic building blocks, according to Manfred Bayer, a member of the Würzburg group. It might even be possible to weave together photonic molecules to form crystals with “photonic band gaps,” that disallow access to photons of certain frequencies, he says. Eli Yablonovitch, of the University of California at Los Angeles is equally optimistic: “We are embarking on an era in which electromagnetic modes will be engineered to control things like the spontaneous emission of light and lasing.”
–Meher Antia
Meher Antia is a freelance science writer.
References
- J. P. Reithmaier et al., Phys. Rev. Lett. 78, 378 (1997)