Nobel Focus: Current for a Small Charge
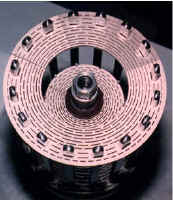
The 1998 Nobel Prize in Physics honors three researchers who discovered and explained a bizarre state of electrons restricted to two dimensions at low temperatures and high magnetic fields. This “fractional quantum Hall” (FQH) state was first reported and then explained by this year’s Nobel Laureates in PRL in the early 1980s. The strangest aspect of the FQH effect is the notion that electric current can be carried in chunks with just a fraction of the charge of an electron, a concept that was fully verified by later experiments. Observations of this and other phenomena in the FQH effect has brought new understanding of the ways in which many electrons can interact in a collective quantum state.
In 1879 Edwin Hall reported the classic effect that bears his name: Running current through a wire perpendicular to a magnetic field causes the electrons to deflect sideways and generate a voltage across the wire’s width. This “Hall voltage” increases continuously with increasing magnetic field, but a century later physicists discovered that under extreme conditions the Hall effect changes character, in what is now called the integer quantum Hall effect. With electrons trapped in a thin layer of semiconductor at low temperatures and high magnetic fields, the Hall voltage reaches a series of plateaus at precise values as the field is increased. Within these plateaus the ratio of the electric current to the Hall voltage (the Hall conductance) is an integer multiple of a conductance quantum, which is determined only by fundamental constants of nature.
Daniel Tsui, now of Princeton University, Horst Störmer, now of Columbia University, and Arthur Gossard, now of the University of California at Santa Barbara, measured the Hall effect for even stronger magnetic fields than previous researchers had used (over 20 tesla). They were surprised to find additional plateaus in the Hall conductance at noninteger values of 1/3 and 2/3 times the basic conductance unit. Although they suggested an incorrect explanation, the team correctly pointed out that their results might imply charge carriers with one-third the charge of an electron.
The integer quantum Hall effect had been explained by assuming independent electrons that did not interact with one another, but the FQH effect required a “many-electron wave function”–a mathematical description of a single quantum state which included all of the electrons in the system. In 1983 Robert Laughlin, now of Stanford University, provided that wave function and showed that it led to fractionally charged “quasiparticles” and was consistent with the other data. Laughlin’s theory of the FQH state was interpreted by others as a type of Bose-Einstein condensation, the same phenomenon that allows electron pairs in a superconductor or helium atoms in superfluid helium to coalesce into a single macroscopic ground state.
From the point-of-view of a single electron, Laughlin’s many-electron wave function has zeroes–called vortices–at the locations of each of the other electrons, but the number of vortices increases with the strength of the magnetic field and can exceed the number of electrons. Although lone electrons cannot normally reside in the same quantum state (they are fermions), when the field is such that each electron is “bound” near an odd number of vortices, the composite objects act like bosons, particles that can occupy the same state. If the field is increased just a bit above one of these stable arrangements–say, when there are three vortices per electron–the sea of electrons moves away from the newly created vortex, trying to maintain the same electron-to-vortex ratio everywhere else. This leaves behind a depleted region with exactly one-third of an electronic charge.
Researchers have continued to study a wide range of phenomena in FQH systems, including the interactions of the fractionally charged quasiparticles themselves. The FQH effect “had a profound effect on our thinking about electronic properties of solids,” says Charles Kane of the University of Pennsylvania. “The idea that you could get these very strange [fractionally charged] objects emerging out of the collective behavior was very new.”