Highest Resolution Images of a Crystal
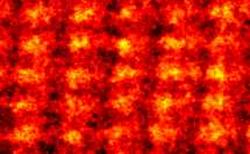
Focusing electron beams like light rays, typical transmission electron microscopes (TEMs) can image individual atoms as close as 1.6 Å apart. But even that resolution does not reach the inherent quantum mechanical limits–the current limitation comes from imperfections in the electron focusing and other technical effects. According to the 9 November PRL, TEM resolution can be doubled by correctly taking advantage of “incoherent TEM,” a method traditionally used for low resolution work. The authors recorded the highest resolution microscope images ever made of crystal structures, showing they can resolve atoms in a CdTe crystal less than 1 Å apart and that even higher resolution images are possible.
Although physicists often use x rays to resolve crystal structures, x-ray methods cannot reveal the structure of atomic-scale defects or individual nanocrystals in a collection of randomly oriented nanocrystals. Instead, x-ray diffraction data reflect average properties over a relatively large region of a sample. Researchers would like to improve TEM resolution to allow higher precision measurements and resolve the most closely-spaced atoms in crystal structures.
A TEM works very much like a conventional light microscope: The beam of electrons is sent through the sample and focused (using magnetic fields as the “lens”) onto a detector to produce the image. But there are two major types of blurring, or “aberrations,” that prevent TEM from reaching higher resolutions. Spherical aberration is caused by the more widely scattered electrons coming to a focal point closer to the lens than those that are scattered at more shallow angles. Ideally, all electrons should focus at the same place. “It’s like trying to do light microscopy through the bottom of a wine bottle,” says Peter Nellist, of the University of Birmingham in England. Chromatic aberration, the other major distortion, is caused by the faster (shorter wavelength) electrons focusing at a different position from the slower ones, a problem that only a perfect electron beam could solve completely.
High-resolution images are normally created with coherent TEM, in which the source is equivalent to a laser beam, but Nellist and Stephen Pennycook of Oak Ridge National Laboratory wanted to push the limits of incoherent TEM, a technique equivalent to illuminating the sample with a lightbulb. Researchers can compensate for spherical aberration to some extent by “underfocusing”–deliberately sacrificing the some of the larger-scale information to get the fine details–but in coherent TEM the improvement is only slight because of chromatic aberration. When Nellist tried the compensation scheme with the incoherent instrument, “I was amazed at the resolution I’d managed to achieve,” he recalls. As he later calculated and now describes in PRL, not only is compensation for spherical aberration easier with incoherent TEM compared with coherent TEM, but the remaining chromatic aberration is also much less severe. It turns out that the incoherent electron waves, which are in random phases, cancel out some of the worst effects that are normally seen with coherent TEM. Nellist and Pennycook demonstrate the best resolution ever seen with TEM, resolving cadmium and tellurium atoms only 0.93 Å apart in a crystal and a sharpened image of silicon corresponding to 0.78 Å resolution.
“It’s very important,” says Ondrej Krivanek, of the University of Washington in Seattle. He says 99% of high-resolution electron microscopy is done with the coherent technique, but that method has essentially reached its resolution limits. This paper should generate greater interest in incoherent TEM, according to Krivanek, particularly as correction lenses, which he is developing, eventually eliminate spherical aberration entirely.