Electrons Carried by Sound Waves
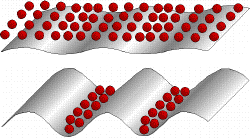
Like miners trapping gold nuggets in a sluice box, physicists can trap electrons between sound waves in a solid. As the sound waves move, this creates a novel way of transporting electrons–and therefore electric signals–from one place to another in a semiconductor. In the 8 March PRL researchers report a dramatic improvement in this method of moving electrons. Possible applications for the technology include CCD cameras, where the charge residing in each pixel needs to be transported to a readout device. In present-day CCD cameras, the charges are transported by a complicated system of electric gates; using sound waves instead might make the devices simpler to fabricate.
The idea of “acoustic charge transport” is over twenty years old, having been demonstrated for the first time in silicon in 1976. However, according to Achim Wixforth of the University of Munich, in the past sound waves have not been very effective in trapping and guiding electrons because much of the sound energy was wasted. As he explains it, the voltage created by the sound waves in the semiconductor creates the equivalent of a corrugated surface at the bottom of a shallow pool of electrons. The motion of the sound waves causes the corrugation to move along the bottom of the pool. If the voltage is too small–that is, if the corrugations are not very deep–very few of the electrons will be dragged along, and they will easily slosh from one trough to the next. So most of the power of the sound wave is dissipated in the electron pool.
Wixforth and his collaborators have now found a way to greatly enhance the voltage (or depth of the corrugations), so that the electrons are completely trapped between the waves, forming strips of charge. Now the strips move in lockstep with the sound waves, and hardly any power is lost by sloshing. To accomplish this, they attached an ultra-thin layer of semiconducting material to the top of a piezoelectric base made of lithium niobate. Like a quartz crystal in a radio, lithium niobate turns mechanical vibrations into strong electric fields. When the researchers vibrated the base, the electromagnetic waves passed through the semiconducting layer as well. They were much more intense than any that have been produced by vibrating a semiconductor alone, and thus were more effective at trapping and shepherding electrons through the material. As a result, Wixforth and his colleagues were able to reduce the power loss by more than a factor of 40.
Other experts on surface acoustic waves are impressed. “Theoretically, you can expect that if a powerful surface wave is launched, the electrons could break up into charged strips,” says Gregory Aizin of the City University of New York. “The problem is how to realize this experimentally. It’s wonderful that they were able to do this.”
–Dana Mackenzie
Dana Mackenzie is a freelance science writer.