Fountain Clock Keeps Good Time
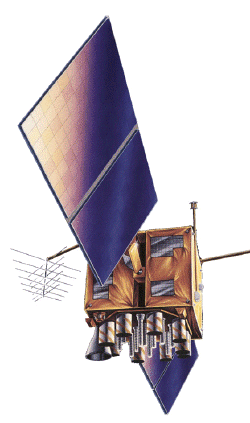
The best clocks in the world are closely tied to the international definition of the second, which is based on a specific transition in cesium atoms. Commercial atomic clocks use a beam of these atoms, but several other technologies are in development in labs worldwide as researchers work toward the next generation of super-accurate clocks. The 7 June PRL describes the best combination of accuracy and stability achieved to date–a cesium clock so stable that only the fundamental laws of quantum mechanics limit its performance, as all other technical sources of instability have been reduced to a lower level.
In a cesium beam clock, the atoms are hit by microwave photons twice during their trip through a long tube–once near the entrance and again near the exit–after which laser probes determine the number of atoms that made a transition from the ground state to the excited state. The frequency of the microwaves is continually adjusted by a feedback loop so that exactly half of the atoms make the transition. (The feedback is more sensitive at this “off-resonance” frequency than it would be if the microwaves were tuned to excite all of the atoms.) A stable clock has this microwave frequency tightly “locked” to the cesium transition, with little variation over time.
Andre Clairon of the Paris Observatory and his colleagues have been perfecting one of the latest generation of clocks, which uses an atomic “fountain,” rather than a beam. The system cools a cloud of cesium atoms to about 1 µK and launches it upwards, so that it passes through a microwave cavity on its way up and again as it drops back down under gravity. The time between microwave interactions is about half a second, allowing for much more precise frequency locking than beam clocks, where atoms reach the second microwave cavity only milliseconds after the first.
Clairon and his colleagues have now reduced the frequency fluctuations of their clock to below one part in for a single fountain cycle–a clock that might lose a second in ten million years. That’s the best stability in the world for a clock that is also extremely accurate, in that it tracks the definition of a second to one part in . Team member Giorgio Santarelli explains that the stability is limited only by the statistics of atomic transitions: The number of atoms excited in any specific fountain cycle will usually be slightly more or less than half, even when the microwave frequency is perfectly tuned, just as one million coin flips rarely yield exactly 500,000 heads.
According to Santarelli, the team achieved the high stability by using a very stable, liquid helium cooled microwave source based on sapphire, rather than quartz. They also made several “tweaks” to the detection system. “It’s like cooking,” says Santarelli. “It depends on how you tune things.” The unprecedented stability will help the team improve their clock’s accuracy because they can now characterize with higher precision the ways in which their microwave frequency differs from that of ideal atoms in a vacuum, upon which the definition of the second is based.
Kurt Gibble of Yale University says that Clairon and his colleagues have “really beautiful data” and that their fivefold improvement over previous clocks is significant. He says the high stability will help them push to the limits of accuracy allowable by a fountain clock using a single cloud of atoms–future clocks may “juggle” more than one cloud and perform even better.