Atoms as Movie Stars
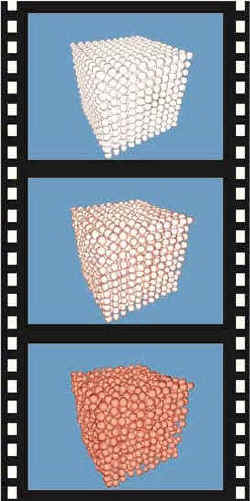
Physicists learned long ago how to find the positions of atoms in a crystal using x rays; today their scientific heirs want to turn those still photos into movies. They dream of watching chemical reactions unfold atom-by-atom and studying the intricate dances of large protein molecules in action. In the 3 January PRL a team describes a step toward those dreams: They directly observed the “wobbling” of atoms on the picosecond timescale following a massive “kick” from a laser pulse. Their experiments demonstrate a way of measuring very high frequency vibrations in solids, which is important for understanding materials at the most basic level. They also found that the atoms don’t respond to the kick as expected.
Although ultrafast laser techniques–recently highlighted by the 1999 chemistry Nobel Prize–allow researchers to follow the action of electrons, visible light can’t detect the positions of nuclei. To make atom-scale x-ray movies, it takes a camera fast enough to capture atomic motions. Roger Falcone of the University of California at Berkeley and his colleagues developed one of the fastest x-ray cameras in the world, according to his graduate student Aaron Lindenberg. The detector’s 3 ps “shutter speed” requires plenty of light, so the team used x rays from the Advanced Light Source at the Lawrence Berkeley National Laboratory.
Their plan was to blast a crystal of InSb with powerful, 150 fs pulses of near infrared light and watch the x-ray diffraction pattern disappear as the atoms were violently forced from their well-ordered positions. “Instead, we saw these big oscillations in the diffracted intensity,” recalls Lindenberg. The oscillations were a direct signal of the atoms collectively ringing like a bell following the laser pulse, which wasn’t quite strong enough to melt the crystal. The oscillating intensity was the most direct observation yet seen of such atomic motions. By changing the angle of the crystal with respect to the incident x rays, which were monochromatic, the team could watch the ringing of different vibrational modes, or “phonons,” having periods from 10 to 40 ps.
When the researchers turned up their laser power even higher–high enough to melt the crystal this time–the diffraction signal disappeared, but not at a uniform rate: Depending on the angle they looked, the signal took anywhere from 20 ps to less than 3 ps to drop away, implying that each vibrational mode made one final collective swing in one direction before becoming disordered, just as a bungee chord might not spring back if it’s overstretched. This behavior was a surprise because the most common models of melting a crystal involve random motion of the atoms, which would destroy the diffraction at a fast and uniform rate.
Lindenberg says the new technique allows new types of measurements of vibrations in solids under extreme stress, a way of directly “mapping” the phonon properties by watching atoms collectively wobble. Such data can be used to test fundamental theories of the solid state.
Andrea Cavalleri of the University of California at San Diego (UCSD) agrees that the experiments convincingly demonstrate an important technique, especially because it reaches phonon frequencies as high as 100 GHz, much higher than any previous methods. He says the highest frequency phonons are the most productive for studies of rapid changes in atomic-scale structure of solids because they have the shortest wavelengths. UCSD’s Craig Siders looks at the long-term goals: “This work will be a landmark on a road which may ultimately lead to the observation and understanding of the biochemical and biomechanical machinery of life.”