Chipping Away at Hardness
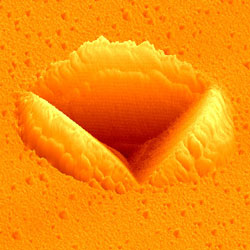
Predicting the hardness of materials based on their atomic structure has often been like trying to scratch diamond with chalk. A characteristic of atomic bonds called ionicity seems to be associated with hardness, and now in the 4 July PRL a team comes up with an explicit formula for hardness based on this property. It successfully predicts the hardness for several materials, including a recently-synthesized superhard material. The result could help establish a microscopic model of hardness and aid in the hunt for new superhard compounds.
Hardness is the ability of one material to resist being scratched or dented by another. This property is not so easily defined at the atomic scale, and there is no fundamental theory that could tell materials scientists how to arrange atoms to make a hard structure. Still, several researchers have come up with different schemes for predicting hardness. They have had some success, but problems remain.
Ionicity is related to the strength of atomic bonds. In a so-called covalent material, such as diamond, germanium, or silicon, each pair of atoms shares a pair of electrons equally to form a bond. In polar covalent materials, there is some asymmetry, and one atom or the other has greater control over any shared electrons. In the extreme case–an ionic material–one atom takes over its neighbor’s electron entirely, and the two are held together by their newly acquired opposing charges. This electrostatic attraction, called an ionic bond, is much weaker than a covalent bond of shared electrons. Ionicity is a measure of the degree of sharing: covalent bonds have the lowest ionicity, and ionic bonds have the highest.
Yongjun Tian and Faming Gao of Yanshan University in Qinhuangdao, China, and their colleagues, focused on covalent and polar covalent materials. They started by assuming that hardness measures the combined resistance of chemical bonds to indentation–the more bonds in a region of the surface, the harder the material. Shorter and denser chemical bonds should then favor hardness. Hard materials should also have low ionicity, the team reasoned, in agreement with other researchers, because covalent bonds are stronger than ionic bonds.
Combining these principles with a 30-year-old theory that describes material deformation in terms of electrons, Tian and Gao came up with a formula for hardness based on ionicity, bond length, and the number of electrons available for bonding. Using the known properties of 11 materials, including diamond, and they found the optimal values for two parameters in the equation.
The final equation predicted (within about 10% accuracy) the measured hardness values for 14 hard oxides, semiconductors, and other pure and polar covalent materials. The team also calculated a likely atomic structure for the superhard compound and found that its predicted hardness matched the observed value. It’s atomic structure hasn’t yet been determined experimentally.
“It appears to be a powerful and useful technique for predicting materials hardness,” says Julien Haines of the Montpellier University of Science and Technology in France. The authors unified several insights “in a fairly elegant way,” says Gerbrand Ceder of the Massachusetts Institute of Technology in Cambridge, but he would like to have seen the model tested against more materials, especially metals, which are a bigger challenge. Still, he says, “every time you can make a connection between a macroscopic property and calculatable things, it’s a step forward.”
–JR Minkel
JR Minkel is a freelance science writer in New York City.