Ultracool Atoms in a Quantum Cavity
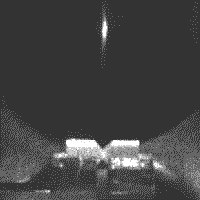
A small cavity made of near-perfect mirrors can trap a photon whose frequency is in tune with the cavity. Adding a single atom to the mix can produce a coherent quantum system in which the photon strongly couples the atom to the cavity. In the 11 May PRL, a team from the California Institute of Technology reports on its studies of single atom-photon interactions inside such a cavity, including possibly the first evidence of forces that could create a kind of atom-cavity “molecule.”
Over the past six years or so, several research teams have studied beams of atoms passing through cavities. The Caltech group’s trick, which it introduced in 1996, is to cool its cesium atoms to 20 microkelvin in a magneto-optical trap (MOT) before releasing them to fall between the curved mirrors of the cavity. With such cold, slow moving atoms, the atom-cavity interaction lasts much longer, and the interaction energy is greater than the atoms’ kinetic energy.
A weak probe laser shines through the cavity axis, and each atom that drops through shows up as a brief, well-defined disturbance in the detected laser intensity. The probe beam is so weak that there is rarely more than one probe photon in the cavity at any moment, but the team has achieved such ideal conditions that they can measure the cavity-atom coupling accurately during the passage of a single atom. By contrast, researchers using atomic beams have had to average over many atoms to measure similar effects.
The Caltech group studied how the signal varied when the laser was detuned, that is, when its frequency was shifted away from the cavity resonance, which was tuned to a cesium transition. They saw a suggestive asymmetry between the signals for red and blue (lower and higher frequency) detunings of the laser. The group speculates that the asymmetry comes from mechanical light forces, a kind of “photon-covalent bonding” between the atom and the cavity. With a single red detuned photon of the right frequency present, the atom sees an attractive potential in the cavity, just as one atom can be attracted to another through the sharing of an electron pair. A blue detuned photon, on the other hand, causes a repulsion. In the words of Christina Hood, a graduate student on the research team, the experiment, with its control of quantum dynamics, “helps us move toward the next wave of engineering: being able to design, prepare, monitor, and manipulate individual quantum states.”
–Graham P. Collins
Graham Collins is a freelance science writer.