Nobel Focus: Neutrino and X-ray Vision
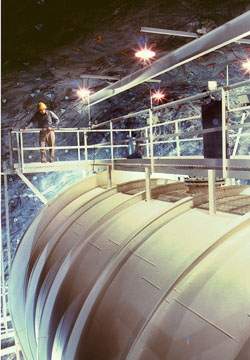
This year’s Nobel Prize in Physics went to three experimentalists who opened the window on cosmic neutrinos and x rays. Raymond Davis of the University of Pennsylvania in Philadelphia and Masatoshi Koshiba of the University of Tokyo shared half of the Prize for detecting neutrinos emitted from sources beyond Earth, while Riccardo Giacconi of Associated Universities, Inc., in Washington, DC, claimed the other half for the discovery of cosmic x rays. Their pioneering work was documented in PRL.
The neutrino, a product of certain nuclear decay reactions, is maddeningly hard to detect, even from the nearby Sun. In the early 1950s, at Brookhaven National Laboratory in Upton, New York, Raymond Davis developed a system for capturing the radioactive argon atoms produced when neutrinos collide with molecules containing chlorine and carbon. He filled a tank in the Homestake Gold Mine in South Dakota with over 600 tons of the dry-cleaning fluid perchloroethylene bubbling helium through it to remove the argon produced by neutrinos. The amount of argon then gave a measure of the number of neutrinos that hit the tank.
In 1968, Davis and his collaborators reported their detection of solar neutrinos, the first direct evidence for the nuclear reactions that power the sun. The solar neutrino flux they measured was several times lower than the first calculations by John Bahcall, now at the Institute for Advanced Study in Princeton, New Jersey, predicted–a discrepancy dubbed the “solar neutrino problem.”
Inspired by Davis’s results, Masatoshi Koshiba converted his underground Kamiokande experiment from a proton decay detector to a neutrino spotter in 1986. Koshiba and his colleagues had placed photomultiplier tubes, which detect single photons, around 2,000 tons of water to see the Cherenkov light that would appear if a proton decayed. Neutrinos bouncing off of electrons would also produce Cherenkov radiation at lower energies, so Koshiba modified the design. Besides confirming the solar neutrino deficit, the experiment detected a neutrino burst from supernova 1987A in the Large Magellanic Cloud, 170,000 light years away. That signal, which was also picked up by another neutrino facility, was the first clear detection of neutrinos from outside the solar system.
Davis and Koshiba made extraordinary contributions in part because “solar neutrino experiments have a sensitivity that is not accessible [with neutrinos] from the Earth,” says Bahcall. In 1998, the Super-Kamiokande experiment, also designed by Koshiba, found strong evidence that the solar neutrino problem was a result of neutrinos oscillating among their three flavors, only one of which was detected [1]. The Sudbury Neutrino Observatory in Canada greatly strengthened that conclusion this year [2].
Riccardo Giacconi had a stubborn quarry of his own. The Earth’s upper atmosphere mostly absorbs high energy cosmic x rays, which are intrinsically hard to focus into usable images. In 1962, Giacconi and his group, then at the private company American Science and Engineering, sent up a rocket equipped with Geiger counters to look for solar x rays reflected from the moon. Instead they found a strong cosmic x-ray source, later named Scorpius X-1, and a uniform haze of x rays across space. Scorpius X-1 was a surprise because it produced far more x rays than visible light.
Resolution of x-ray signals gradually improved, and the use of satellites increased observation times. Giacconi’s group led the way in space-based observing with UHURU in 1970 and continued with the Einstein Observatory in 1979. The two missions each revolutionized x-ray astronomy, says Fred Lamb of the University of Illinois at Urbana-Champaign. Thousands of x-ray sources are now known, from clouds of hot gas to binary stars and other dense stellar objects, such as black holes. “The ‘62 flight alone, believe me, is worth a Nobel Prize,” says Walter Lewin of the Massachusetts Institute of Technology in Cambridge. Lamb is pleased that this year’s Prize honors astrophysics and astronomy. “It’s great to see this kind of work recognized,” he says.
–JR Minkel
JR Minkel is a freelance science writer in New York City.
References
- Y. Fukuda, et al.,mPhys. Rev. Lett. 81, 1562 (1998) .
- Q. R. Ahmed, et al., Q. R. Ahmed et al.Phys. Rev. Lett. 89, 011301 (2002);, Phys. Rev. Lett. 89, 011302 (2002)