Bucky Diamonds In The Rough
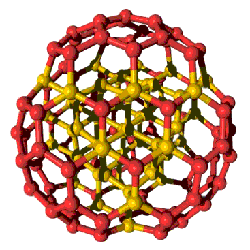
Nanometer-size diamonds could have a buckyball-like shape, prompting researchers to coin a new term: “bucky diamonds.” Both diamonds and the soccer-ball-shaped cage molecules called buckyballs are made of pure carbon, and according to the 24 January print issue of PRL, nanoscale diamonds could surround themselves with buckyball shells. But several experts in the field are not convinced by the data. If the work is confirmed, this new family of carbon clusters may provide new insights for the development of optoelectronics–futuristic devices that process both light and electrical signals.
Experts have speculated that nanodiamond films could be used as heat-tolerant semiconductors or as part of flat panel displays. These films are made by depositing layers of carbon atoms on a surface, but single nanodiamonds are forged in an explosion of TNT, the explosive hexogen, and water. While researchers know the properties of macroscopic diamonds, no one has been able to study the structure of these molecule-sized diamond particles.
Jean-Yves Raty, then at the Lawrence Livermore National Lab in California, and his colleagues modeled the structure of the particles on a computer. These simulations suggested that as the particles get smaller, the structure becomes somewhat unstable. The diamond core remains intact, but the surface breaks into carbon hexagons and pentagons, effectively encasing the diamond in a shell similar to a buckyball, which is also called a fullerene.
To test whether such structures could exist in the real world, the team purchased a batch of nanodiamonds that had been created with high explosives and hit the particles with x rays. The absorption spectra showed signatures that were consistent with the buckyball shape.
In other semiconductors such as silicon, nanoscale particles of the material can emit light at a wavelength determined by the size, rather than the material. The effect is called quantum confinement, and it only occurs when the electrons and holes are limited to small regions. Raty and his colleagues calculated that quantum confinement doesn’t exist in nanodiamonds, even down to sizes as small as two nanometers across. This result suggests that researchers can’t rely on past experience with semiconductors to predict diamond’s behavior, Raty says. Since he and his colleagues believe that nanodiamond films are merely clusters of bucky diamonds, the findings could prove important as researchers try to construct diamond electronics.
Tyrone Daulton of NASA’s Stennis Space Center in Mississippi is skeptical. The authors “did not prove that a fullerene-type structure is the only structure” that could have produced the x-ray signatures, he says. He confirms, however, that nanodiamonds may one day prove important for technological applications. Dieter Gruen of Argonne National Lab in Illinois feels that the results are intriguing, but speculative. And Jim Davidson of Vanderbilt University in Nashville, Tennessee, points out that the structure of the material used in applications can differ from that of the nanoparticles. “To use nanoparticles, they must somehow be coalesced, suspended, or interconnected, which can drastically change their ‘stand alone’ properties,” he says.
Livermore team member Giulia Galli says that “although we do not have an absolute proof of the structure, many experimental results could already be explained” by it. She says the simulations “put a challenge out there to the experimentalist and theorist alike to confirm the existence of such a structure.”
–Pam Frost Gorder
Pam Frost Gorder is a freelance science writer in Columbus, OH.