Craters in a Sandbox
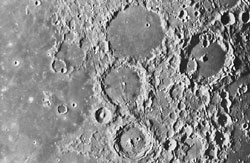
Large lunar craters have stories to tell about explosive collisions of meteorites with the moon. But to interpret them fully, researchers need to learn in detail how they are created. Two teams have discovered that balls dropped into sand and other granular matter form craters remarkably similar to those on the moon. In the 16 May PRL, one team reports the relationships between a colliding object’s energy and the diameter and depth of impact craters. A second team, reporting in the 4 September PRL, recreated a variety of lunar crater shapes and examined the relationship between shape and energy of impact.
Some researchers have attempted to model meteorite impacts with explosive charges set in the ground, but the craters formed don’t have the more complex features of large lunar craters. Still, geophysicists have developed a rule of thumb that both crater diameter and depth increase with impact energy according to a formula where energy is raised to the 1/4 power. Even so, says Douglas Durian of the University of California at Los Angeles (UCLA), the conventional theory doesn’t give much insight into how the ground reacts to an impact, nor into what creates the distinctive crater shapes.
John de Bruyn, of Memorial University of Newfoundland in St. John’s, Canada, says that his group wasn’t originally interested in craters at all, but was considering the crown-shaped splash that appears when an object is dropped into a liquid. “We had just acquired a high-speed camera, and my post-doc was interested in seeing crown splashes in sand.” It turned out that sand doesn’t splash like a liquid, but the resulting pits looked a lot like craters on the moon.
De Bruyn’s group dropped a steel ball into a container of glass beads of various sizes. They found that the geophysical rule of thumb for depth and diameter was approximately true, even though glass is nothing like moon rock, and the steel ball was hitting with much less energy than a meteorite. The team also showed that their crater structures were very similar to lunar craters. They created simple bowls with raised rims, bowls with small mountains in the middle, and bowls with rings around them, depending on the impact energy and bead size. The team now wants to learn in more detail how a variety of factors influence crater shape.
Durian and his colleagues dropped balls of many different materials and densities–from silicone rubber to ceramic–into materials such as sand, popcorn, and ice cream sprinkles. But the materials didn’t matter much; the depth was mainly affected by the density and diameter of the ball and the height from which it was dropped. Their crater diameters followed the “energy to the 1/4 power” rule of thumb, but the depth didn’t depend on energy in a simple way. This result doesn’t contradict the work of de Bruyn’s team because the UCLA researchers defined depth by measuring to the bottom of the buried ball, which was deeper than the bottom of the crater.
An old theory predicts a depth exponent of 1/4 by assuming that most of the meteorite’s energy goes into lifting grains out of the crater space. But Durian suspects that granular materials can dissipate energy in a different way. At the early moments after impact, the grains may “seize up” and react like a solid mass before allowing the object to penetrate moments later. The same effect prevents you from slapping your open hand down through sand at the beach, even though it yields with a gentler push. Durian says that a better theory would account for this property and might agree more closely with his team’s depth data.
“There is surely a great deal of internal energy dissipation that does not go into just lifting particles,” says Robert Behringer of Duke University in Durham, North Carolina. He thinks Durian’s paper is “potentially important.” Lev Tsimring of the University of California at San Diego says that the two papers give him hope that researchers can “understand geological events by doing small-scale experiments and relatively simple simulations.”
–Kim Krieger
Kim Krieger is a freelance science writer in Norwalk, Connecticut.
More Information
Related work by Durian and K.A. Newhall studying crater depth: Phys. Rev. E 68 060301(R) (2003)