Don’t Let Them Know You’re Watching
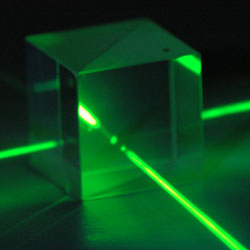
Quantum mechanics says that two distant particles can be intimately connected, so that they affect each other even when they’re far apart. In the 7 May PRL, researchers report measuring a similar effect for a single particle that was in two places at once. The trick is to measure the particle’s properties in each place without knowing whether it’s there. The results may suggest new ways to make quantum computers.
In the past researchers have explored the surprising predictions of quantum mechanics using pairs of particles. Many experiments have shown that so-called entangled quantum particles can communicate even when separated by a large distance. These long-distance connections are an example of a strange quantum property called nonlocality.
Now Björn Hessmo and his team from the Royal Institute of Technology in Kista, Sweden, have measured nonlocality for a single particle of light, a photon, adapting a method proposed in the early 1990’s [1]. Hessmo’s team sent a light beam into a standard beam splitter to deflect half of the light to the left and let the other half pass straight through. They used a beam so dim that only one of its photons was in the setup at a time. At the ends of the two light paths, or “arms” of their setup, they placed photon detectors.
According to quantum mechanics, a single photon passing through a beam splitter takes both the reflected and transmitted paths simultaneously. It remains in both paths until it’s been detected in one place or the other. But to prove that the photon was in both arms at once, the researchers needed to somehow note its effect without explicitly detecting it–otherwise they would spoil the nonlocality. So they shined a second, “reference” light beam into the same beam splitter, which sent it along the same two paths. The detectors couldn’t distinguish the original photon from the reference beam’s photons.
To show that the single photon was present in both arms, the researchers monitored its effect on the reference beam. When the two beams in one arm were “in phase”–meaning their oscillating electric fields were synchronized–they produced more photons in that arm’s detector than when they were out of phase. But this enhancement also extended to the other arm’s detector because “part” of the photon existed there, too. So when the single photon was detected in one arm, it was more likely that a reference beam photon was detected in the other arm at the same time–a photon “coincidence.”
The researchers adjusted the phase of the reference beam using a device located in one arm. When the beams were in phase, they detected five times as many coincidences as when they were out of phase.
Hessmo says that the stability of this new scheme makes more complex arrangements seem feasible, including ways of harnessing the laws of quantum mechanics to do superfast calculations, or “quantum computing.” Lucien Hardy of the Perimeter Institute in Waterloo, Ontario, who helped define the strategy to measure a single photon’s nonlocality, agrees that this is an “ingenious modification.” He adds that this is “quite a difficult experiment, and I’m impressed that they got such a clean signature.”
–Don Monroe
Don Monroe is a freelance science writer in Murray Hill, New Jersey.
References
- S. M. Tan, D. F. Walls, and M. J. Collett, “Nonlocality of a single photon,” Phys. Rev. Lett. 66, 252 (1991); L. Hardy, "Nonlocality of a Single Photon Revisited" Phys. Rev. Lett. 73, 2279 (1994) (Scroll down to “see also” links for some researchers’ objections to these papers.)
More Information
This experiment is similar in many ways to the classic two-slit interference experiment.
G. J. Pryde et al., “Measuring a photonic qubit without destroying it,” Phys. Rev. Lett. 92, 190402 (2004) demonstrates the measurement of a photon’s polarization without destroying the particle.