Photon Marathon
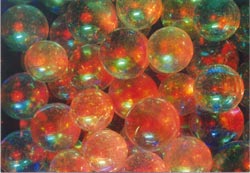
Somewhere between a light bulb and a laser is an unusual and sometimes puzzling type of light source called a random laser. It emits laser-like light, but in all directions. Now a research team believes they understand one of the mysteries of random lasers: why they can emit blasts of monochromatic light as pure as those of an ordinary laser. As the team reports in the 30 July PRL, their experiments and computer simulations show that each pure pulse begins as a single “lucky” photon that manages to bounce around hundreds of times before escaping.
In a typical laser, the light bounces back and forth inside a reflective cavity containing an amplifying material, such as a laser crystal. The ricocheting photons recruit more photons with each pass through the material and can escape through a partially transmitting mirror to make a beam.
Now imagine taking the crystal out of the cavity and grinding it into a powder, and you have a random laser. Hit it with brief pulses of excitation light, and the powder glows in response. The amplification happens when each photon scatters around many times inside the powder, continually eliciting more photons as it travels on a so-called random walk. When it finally escapes the powder, the light usually contains a spread of wavelengths that is broader than the nearly-single-wavelength output of a normal laser.
But if the amplification is strong enough, a random laser spits out extremely monochromatic light at several wavelengths, light that dominates its broader background emission. These “ultra-narrow” spikes in the spectrum appear at different wavelengths with each pulse and are unpredictable, unlike a normal laser, where the size of the reflecting cavity determines the beam’s wavelength. Some researchers have proposed theories to explain this phenomenon, but others have remained unconvinced.
Now Diederik Wiersma of the European Laboratory for Nonlinear Spectroscopy in Florence and the Italian National Institute for the Physics of Matter and his colleagues have come up with an explanation, using results from experiments and computer simulations. The team’s random laser consisted of a laser dye dissolved in alcohol along with particles of zinc oxide to scatter photons. When excited with green laser pulses, the mixture emitted the narrow peaks that others had seen.
Computer simulations reproduced the results and led to the explanation, which the researchers call the “lucky photon” effect. Although most photons emerge from their random walks through the liquid after about ten “ricochets,” a very few photons walk the photon equivalent of a marathon–from 100 to 1,000 ricochets. In these rare cases, the original photon picks up an enormous number of companion photons of precisely its wavelength–perhaps of them in the case of a photon that ricochets 500 times.
“Why didn’t we think of that before?” Wiersma recalls wondering after he and his colleagues ran the simulations. The random laser problem is deceptively similar to the more common scattering problems physicists look at, in which the medium doesn’t amplify. Without amplification, a single photon traveling a marathon path length doesn’t make a difference. “Once you understand that it happens, it is quite a natural explanation,” says Wiersma.
“For sure, this model explains the random laser effect in some of the systems,” agrees Mikhail Noginov of Norfolk State University in Virginia, noting the “good agreement” between the numerical calculations and experimental results. He hopes future experiments will test how broadly the model applies to other types of random lasers.
–Chelsea Wald