Putting the Reins on Light
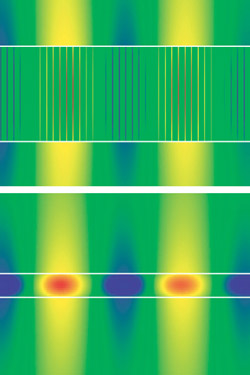
Light slows down when it enters a medium such as glass or water, and its new speed depends on the material. In the 20 May PRL, however, researchers calculate that they can control the speed of light in a new way. They found that a series of metal strips, with gaps between them, can act somewhat like a uniform slab of glass, except that the speed of light depends on the dimensions of the strips rather than on the material. By narrowing the gaps, they propose making a structure that slows light much more than any conventional material can. Such structures could help make tiny optical or optoelectronic devices both smaller and more efficient.
Since the surprising result of the late 1990’s–that long-wavelength waves can penetrate a slotted sheet of metal [1]–researchers have been investigating the curious interactions of electromagnetic waves with similar structures. Jung-Tsung Shen, Peter Catrysse, and Shanhui Fan of Stanford University in California imagined a series of metal strips stacked somewhat like the slats of a Venetian blind positioned to let in daylight, except with strips fatter than the spaces between them. They used classical electromagnetic theory to calculate what happens when waves encounter the structure. Even though electric fields can’t exist inside the metal, waves whose magnetic field is parallel to the slats can set up an electromagnetic disturbance that propagates through the narrow gaps, in effect reconstituting the incident waves on the other side of the structure. Shen and his colleagues found that if the wavelength is much longer than the gap size, the “blind” acts almost exactly like a slab of glass with an extra-slow speed for light propagation.
The light speed depends only on the relative dimensions of slats and gaps, not on the materials used. It can therefore go much lower than the values obtainable in commonly used optical materials, such as gallium arsenide and indium phosphide, Shen says.
The Stanford researchers were further surprised, Shen adds, to find a second kind of wave propagation in the structure. In what they call a waveguide mode, the Stanford team found that waves will travel laterally through the structure, with the light energy propagating from one gap to the next. Here again the gaps sustain the oscillating fields, which spread out beyond the structure, allowing the gap-to-gap communication. In this case, too, the structure behaves like a slab of transparent material.
Because real metals do not have perfect electrical conductivity, as the researchers assumed in their analysis, an actual series of slats will show small additional effects not revealed by the team’s calculations, Shen says. But the underlying equivalence will hold true, he adds, and will simplify the design of devices with such structures. A structure that mimics a material with extreme optical properties could allow some devices to be made smaller than conventional materials would allow. There would also be smaller leakage of light at the interface between such a structure and an ordinary material.
These results are an interesting demonstration of the ability to make an effectively new material out of existing ones, says Steven Johnson of MIT. One limitation of this structure, he points out, is that for incoming waves oriented in ways that aren’t perfectly transmitted, it acts like an ordinary metal. On the other hand, he speculates, it might be possible to broaden its applicability by creating more complex geometries.
–David Lindley
David Lindley is a freelance science writer in Alexandria, Virginia.
References
- J. A. Porto, F. J. García-Vidal, and J. B. Pendry, “Transmission Resonances on Metallic Gratings with Very Narrow Slits,” Phys. Rev. Lett. 83, 2845 (1999); described in Phys. Rev. Focus 4, story 18 (1999)