Unraveling a Twisted Tale
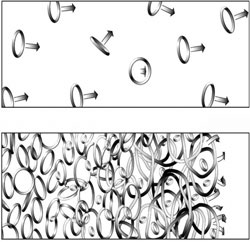
Even a superfluid can get into a tangle. Although certain liquids move without resistance or viscosity, they still break up into turbulent flow patterns when sufficiently agitated. In the 15 July PRL, researchers report the most direct observation yet of the transition from smooth flow to turbulence in a superfluid and confirm a theoretical model of the process proposed by others. The findings may shed light on the complexity of turbulent motion in normal fluids, a phenomenon that has challenged physicists for over a century.
Turbulent fluids show up in oceanography, aviation, pipe flow, and industrial processing, but physicists still have great difficulty describing and studying the constantly changing mix of eddies that constitute turbulent flow. Many have turned to the simpler world of superfluids to look for insights. In a superfluid, atoms can move relative to each other only in restricted ways. Rotation takes the form of atoms circulating about so-called vortex lines, somewhat like the cores of tornadoes. Vortex lines cannot have ends within the superfluid, so they must either extend to the boundaries of the fluid or else curl around to form closed vortex rings, similar to smoke rings. For decades physicists have suspected that quantum turbulence in a superfluid arises when vortex lines and rings become so dense that they interact and tangle with each other, but direct evidence for the transition to turbulent flow has been hard to come by.
Shaun Fisher and his colleagues at Lancaster University, England, sought ways to measure the behavior of vortex rings in a pure superfluid state of liquid helium-3 at temperatures around 100 microkelvin. In these ultracold conditions, the tiny heat content of the liquid is carried by coherent, collective motions of helium-3 atoms known as quasiparticles. More than 15 years ago the Lancaster scientists learned to measure the abundance of quasiparticles by waggling a thin wire loop back and forth in the superfluid. The quasiparticles act as a brake on the motion of the wire, so the greater the resistance the wire feels, the more quasiparticles must be present. By contrast, the quasiparticles bounce off vortex rings and lines, so the more rotation there is in a region, the fewer quasiparticles. The waggling wire thus acts as a kind of camera for rotation, Fisher says, with quasiparticles providing the illumination.
The scientists set up a delicate wire mesh, a few millimeters on each side, in a volume of superfluid helium-3, and vibrated it to agitate the fluid. An adjacent wire measured the density of vortex rings produced. When the mesh was vibrated at low speeds and then switched off, the vortex rings disappeared almost instantly. But for a mesh vibration speed above about 3.5 millimeters per second, the amount of rotation within the fluid instead decayed away exponentially, on a timescale of about 10 seconds.
Consistent with other proposals, the team believes that gentle agitation produces individual vortex rings that fly away from the mesh and don’t interact with each other. Under fiercer agitation, however, vortex rings in greater numbers tangle and hook up with each other and take some time to untangle and disappear. Further evidence for the low-velocity part of this picture came from changes in the behavior of vortex rings as the team varied the temperature.
Makoto Tsubota of Osaka City University, Japan, says this “excellent work” succeeds in showing how individual vortex rings give rise to superfluid turbulence because the Lancaster scientists have learned to produce the rings in such a controlled way. The group “has no peers” in these experimental techniques, he adds.
–David Lindley
David Lindley is a freelance science writer in Alexandria, Virginia.