The Proton’s Strange Magnetism
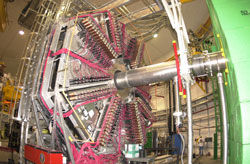
The proton is made of three quarks–two up quarks and one down quark–but other types constantly flit into and out of existence. The 26 August PRL provides the first detailed picture of strange quarks in this mix and indicates that they make a significant contribution to the proton’s properties. About 5% of the proton’s magnetic moment comes from strange quarks, according to the results, although theoretical predictions have been ten times smaller. The discrepancy highlights the serious limitations in the current calculation techniques.
Accompanying the proton’s three permanent quarks are additional quark-antiquark pairs that constantly appear and disappear, including many strange quarks. The heavier charm, bottom, and top quarks don’t appear to any significant extent. At any given time, the fugitive quarks outnumber the three permanent constituents. All of these quarks are electrically charged, so their presence gives the proton an internal structure of charges and currents. Quarks also participate in the so-called weak nuclear interaction, and therefore produce an internal pattern of weak charges.
The G0 collaboration, an international team of over 100 physicists at the Thomas Jefferson National Accelerator Facility in Newport News, Virginia, probed the proton’s interior by bouncing fast-moving electrons off protons in a liquid hydrogen target. The electrons respond predominantly to the proton’s electrical structure, which influences the pattern of electron scattering.
To separate out the effect of the weak interaction within the proton, the team measured a parts-per-million difference in the scattering rate of electrons polarized in two different ways–spins pointing either along the direction of motion or in the opposite direction. Because the electromagnetic interaction is identical for both polarizations, this asymmetry arises purely from the weak interaction, explains G0 spokesman Douglas Beck of the University of Illinois at Urbana-Champaign.
Disentangling the contributions of the three quark types requires a comparison of three independent types of scattering data–akin to three different “maps” of the quarks. Data from many prior experiments have given electromagnetic maps of the proton and also of the neutron, which is identical to the proton if up and down quarks are interchanged. The G0 experiment now provides a map of the proton according to the weak interaction, and this third view permits the strange quarks to be distinguished from the other two.
The results are consistent with a handful of data points from previous experiments but depict the proton’s inner workings over a much wider range of collision conditions. To complete the picture, the G0 team has moved the detector to the other side of their target, to detect electrons that bounce backwards off the target protons. Results from that experiment, scheduled to run through much of 2006, will enable the researchers to separately estimate two quantities that they cannot currently untangle–the strange quark contributions to the proton’s magnetic moment and to the distribution of charge within it.
While G0 doesn’t yet have final results, the data, combined with previous experiments, suggest that the strange contribution to the proton’s magnetic moment is about 5 percent. That value “contradicts most theoretical calculations,” says John W. Negele of the Massachusetts Institute of Technology. One recent paper [1] estimates minus 0.5 percent, for example. Such calculations require either enormous computer power or fairly restrictive simplifying assumptions, so many in the field are not surprised by the glaring discrepancy.
The G0 results “give the first really good picture” of what strange quarks are doing inside the proton, says Negele, and offer a stiff challenge to theorists. But he adds that a new generation of computers, with architecture dedicated to calculating quark interactions, is enabling theorists to tackle problems that until now they had only dreamed about.
–David Lindley
David Lindley is a freelance science writer in Alexandria, Virginia.
References
- D. B. Leinweber, et al., “Precise Determination of the Strangeness Magnetic Moment of the Nucleon,” Phys. Rev. Lett. 94, 212001 (2005)