Cracks in the Theory of Cracks
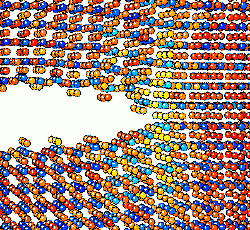
If an airplane engine falls off or a rocket breaks up in flight, cracks in the structures are sometimes to blame. Although physicists understand many electrical and magnetic properties of materials in terms of microscopic theories, the cracking process has proven difficult to describe at the level of individual atoms. In the 10 May PRL, a team describes the first study of crack propagation that can directly test computer models of atomic interactions. The researchers observed cracks propagating through silicon crystals and found that the approximations that they and others use in computer simulations did not agree well with the experiments, suggesting that the approximations miss some of the complexities of the process.
The motion of a crack is difficult to model theoretically, partly because so many different length scales can be involved–from the stress on a large chunk of material down to the “decisions” of precisely which atomic bonds will be broken, and many scales in between. Crack propagation is also a nonequilibrium and nonlinear process–small changes in conditions can lead to large effects–unlike many other processes in which the material is assumed to respond like a simple spring in all directions. Michael Marder of the University of Texas at Austin has spent many years studying crack propagation with computer models, and he wanted to experimentally test some of the approximate interatomic force laws such programs rely upon for reasonable computing speeds.
Marder, his graduate student Jens Hauch, and their colleagues built a pair of rectangular steel frames between which they glued a single-crystal wafer of silicon. Pulling with 8000 N of force along one direction, the frames stretched by 1 µm, enough to split open a smooth crack through the crystal. By carefully preparing a “seed” crack at the wafer’s edge and varying the stretching force, the team could precisely control the energy “fuel” supplied to a crack and measure the speed with which it propagated.
The researchers compared the relationship between energy and speed with results from their atomic-scale computer simulations and found poor agreement, says Marder. “In most cases you could easily tell the difference in fracture energy with your fingers,” he says, if you compared the effort needed to break the real silicon with that needed for the virtual crystal. But regadless of the simulations’ accuracy, Marder says the point was to control crack propagation with such precision that this macroscopic experiment could directly test the atomic-scale force laws used by theorists. “The accomplishment here is getting both the experiment and the simulations on the same graph,” he says.
Robb Thomson of the National Institute of Standards and Technology in Gaithersburg, MD, calls the work “spectacular and unprecedented” because he knows of no other such direct comparison between a fracture experiment and a simulation. The reason, he says, is that silicon is so brittle that its cracks are notoriously difficult to control. On the other hand, silicon is the simplest and most popular material for theoretical studies, so it was important to use silicon for the test.