Counting the Ripples in a Light Wave
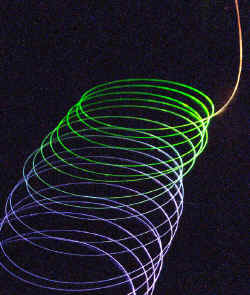
Measuring the frequency of your heartbeat is pretty simple–just count the beats in a given period of time. Even a radio frequency signal at 100 MHz can be measured by an electronic counter in much the same way. But a 600 THz( Hz) light wave oscillates far too fast to count, so precision measurements of optical frequencies have traditionally used huge and cumbersome setups, available only to a handful of labs worldwide. In the 29 May PRL a collaboration of physicists reports they have drastically simplified the process to the point that almost any physics lab could measure optical frequencies nearly as precisely as the standards institutes do. The new technique will lead to new tests of a host of fundamental physics principles.
Measuring things to 12 decimal places is not just boring detail work, maintains John Hall of the National Institute of Standards and Technology (NIST) and the University of Colorado in Boulder. As measurements of the light associated with atomic transitions have become more accurate over the past century, researchers have learned more about the quantum world and the physics of atoms. “It’s like one of those Russian dolls–you just never come to the last one,” says Hall.
In 1983 Hall and his colleagues published the most precise optical frequency measurement at the time, but it took eight physicists and perhaps 20 lasers. Starting from the 9 GHz signal of a cesium atomic clock, which defines the unit of frequency (Hertz), they needed to double and triple the frequencies of various oscillators in a “chain,” to directly connect with the frequency of light to be measured. Carefully comparing each frequency in the chain with the next one allows the equivalent of counting the number of cycles in a light wave each second.
Hall, Ted Hänsch of the Max Planck Institute in Garching, Germany, and their colleagues, have now bridged that gap in one step. The system uses a laser that generates ultrashort light pulses–12 fs, or s in width–at a rate near 100 MHz, which is synchronized with an atomic clock. The spectrum (Fourier transform) of this light is a “comb” of ultranarrow lines spaced every 100 MHz in frequency, over a limited range of light frequencies. The team sends this light through a new type of optical fiber that spreads the reddish pulses over most of the visible range, creating a comb with millions of lines whose spacing is precisely determined by the cesium clock. As compared to, say, tripling a laser frequency to get the third harmonic–about as far as one could go directly in the past–the comb allows access to the four millionth harmonic. “That’s four million stable lasers,” says Hall.
Ideally, the frequency of any laser beam could then be determined by using standard techniques to find the distance in frequency between the laser line and one of the comb lines. The only problem is that, while the comb spacing is precisely known (about 100 MHz), its absolute position is not defined. So the team used the fundamental and second harmonic lines of a neodymium:YAG laser (frequencies exactly a factor of two apart) to get the extra information needed to nail down any optical frequency. In the process, they made the most accurate measurement ever of the YAG frequency, at the level of They also measured two standard laser lines to similar accuracy as proof that the system gives results equal to or better than those of the international standards agencies. Later refinements, published recently in Science, have allowed the team to eliminate the YAG laser from the setup.
Hall foresees a commercial instrument being marketed within five years that will allow any physicist to measure an optical frequency down to the kHz level as easily as they now measure radio frequencies. The technology has already spawned experiments that push theories of fundamental atomic physics to new limits. Leo Hollberg, also of NIST, but not directly involved in the work, says the new technique is “a revolution in frequency measurement” that will likely lead to new super-accurate clocks based on optical frequencies, rather than the microwaves of today’s atomic clocks. Hall expects many unpredictable applications for a device that provides four million stable laser lines at once.