A Knotty DNA Problem
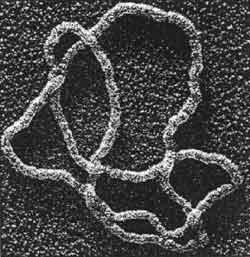
Inside every nucleus of every cell in the body there’s a knotty secret–the DNA is constantly getting tangled up. In the 31 December PRL, a team describes tying knots in a strand of DNA and tracing the knots’ movements along the strand. They found that the DNA slid smoothly through the knots, which allowed the knots to move without friction. The results could help researchers better understand the enzymes that untangle DNA and that might be targets for cancer drugs.
Long strands of DNA floating in a cell’s nucleus can easily become tangled, just as a long extension cord does when left in a heap. Knotted DNA makes it harder for a cell to read genes, but enzymes called topoisomerases can untangle them, if the knots don’t spontaneously unravel from random jiggling.
To study DNA tangles systematically, Robert Bao, Hen Jin Lee, and Stephen Quake, of the California Institute of Technology in Pasadena, submerged a DNA strand in polyethylene glycol, a viscous liquid, to slow down its motion. They then tied knots in it by grabbing the ends with so-called optical tweezers. Each of the two tweezers was a focused laser beam that could grasp a plastic bead glued onto one end of the strand. The DNA, much tinier in cross section than the plastic beads, could pass through the lasers as it was knotted without disrupting the optical tweezers.
The researchers pulled a bit after tying each knot, but they couldn’t pull as tightly as they would a shoelace, thanks to the electrical repulsion between the charges on every part of the strand. These slightly loose knots slid without friction along the strand, so they acted like a mathematician’s idealized knots. The team plotted how knots of various complexities moved along a strand of DNA in time, propelled only by random thermal fluctuations. They found that the movement of the knots agreed with theories of the behavior of ideal knots. The more complex the knot, the slower it slid. The movement of the knots seemed to be closely connected to the serpentine way polymers such as DNA wiggle around obstacles.
Quake and his colleagues say their visible knots of known shapes should be ideal for learning how topoisomerases simplify complex knots inside cells. Knots appear in DNA after replication and other cellular processes, and the team hopes to develop a “dictionary” of knots that result from specific events. Bao adds that one reason he and his colleagues want to study topoisomerases is that disrupting them in cancer cells may be a method of killing tumors.
The work is “exciting,” says chemist Nadrian Seeman of New York University. “A large number of deliberate and specific knotted molecules have been constructed.” He adds that the impact of the work on topoisomerase research is likely to be substantial if the team can keep track of the products of enzyme reactions based on the knots formed.
–Kim Krieger
Kim Krieger is a freelance science writer in Norwalk, Connecticut.