Breaking Single Protein Bonds
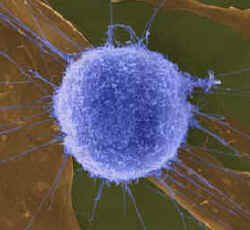
When a white blood cell rolls along a blood vessel wall toward a site of injury, it must attach to that wall with just the right strength: If too tightly bound, it won’t move at all; if too weakly bound, it washes away in the blood stream. Carefully tuned mechanical bonds are also important in muscle fibers and gene transcription in cells. Recent experiments have shown that the strength of a biomolecular bond is not determined simply by the bond energy but also depends on how fast it’s pulled apart, and in the 19 July PRL a team of biophysicists explores a wide range of pulling speeds. They observed the transition between two different regimes of protein bond breaking as predicted by a theory which may apply to many areas in biology where bonds are mechanically stressed.
Tabletop models of molecules make the bonds look static–pull hard enough and the plastic hydrogen atom pops off. But real bonds can break on their own, given enough time, because Brownian motion is always challenging their holding power. The bonds between protein molecules are often meant to be temporary, so “spontaneous” bond breaking can happen in a short time, unlike normal covalent bonds, which last much longer.
To investigate both spontaneous and force-induced bond breaking in the same bond, Rudolf Merkel of the Technical University of Munich and his colleagues looked at the binding of two protein molecules. They used IgG antibody proteins that bind specifically to protein A, which is found on the surfaces of certain bacterial cells, and coated two tiny plastic beads, one with each protein. The team placed one bead at the tip of a micropipette and stuck the other onto a red blood cell which served as a force-measuring spring. By touching the beads together and then pulling them apart at different rates they found the “yield force” at which the bond broke for a wide range of pulling rates, or “loading rates.”
Merkel and his colleagues were able to separate the beads at rates both faster and slower than the rate of spontaneous bond breaking. At fast loading rates they saw force-induced breaking of the bond at relatively high forces, whereas at very low separation rates the bond was often broken with very little force applied because the proteins could separate spontaneously. Although the two regimes had never been observed in the same bond before, previous work suggested that bonds should break spontaneously when stressed slowly enough for Brownian motion to have an effect. As an extra check, they noted the time required for the IgG and protein A to disconnect in the absence of any applied force, and it matched the value expected based on their loading rate data.
Their results seem to confirm a previously proposed model that unifies the two types of bond breaking with one simple theory. Merkel says this theory should help researchers understand cell adhesion phenomena–such as the tearing of tissues which occurs in injuries–because cells are normally held together by the mutual binding of surface proteins.
Steven Block of Princeton University says the work is “an elegant verification” of the idea that a bond’s resistance to force depends on how it’s stressed. He adds that the theory must be quite sound because the molecule-to-molecule variations in structure of the IgG’s the team used did not obscure the main results. Block summarizes the take-home message this way: “If I lean against the Tower of Pisa for long enough, it will eventually fall over.”