Spin-Interaction Studies Take on a New Dimension
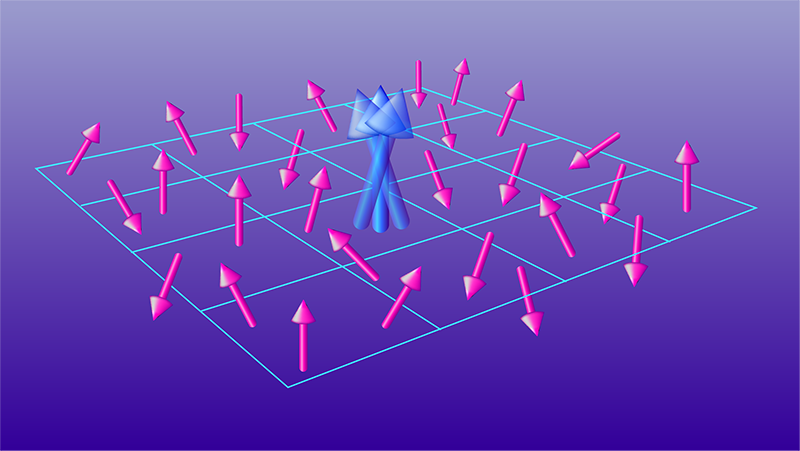
Diamond defects, nitrogen-vacancy (NV) centers in particular, serve as a rich playground for studies of spin physics. Over the past two decades, techniques for manipulating and reading out the quantum states of NVs with optical and microwave radiation have been fine-tuned for applications of these defects as magnetometers and qubits. An important research direction involves understanding and controlling how interactions with the environment can affect the NV’s quantum properties. Now two independent teams—the first led by Nathalie de Leon at Princeton University [1], the second led by Norman Yao at the University of California, Berkeley, and by Ania Jayich at the University of California, Santa Barbara [2]—have addressed. this question for a configuration relevant to multiple applications: an NV center interacting with a 2D ensemble of spins formed by unpaired surface electrons or by impurities engineered within the diamond. The results allowed the teams to develop improved models for describing how these interactions affect the quantum coherence of the NV spin—information that could have profound implications for the use of crystal defects in both quantum metrology and quantum simulation.
Diamond has a number of interesting defect species, with the NV center playing a starring role in optical quantum information applications [3]. An NV is formed by two missing neighboring carbon atoms in the lattice, one of which is replaced by a nitrogen atom. This configuration acts as a two-level spin system that can conveniently be prepared and read out via lasers. An additional control tool is offered by the use of carefully designed microwave pulse sequences that manipulate the populations of those two levels. Such microwave schemes can prepare the NV in spin-state configurations that are optimal for certain tasks—such as probing the magnetic interactions of the NV with its environment [4].
In addition to such tools, both teams exploited diamond-growth techniques continuously refined since diamond NV centers entered the quantum information scene 20 years ago. Such techniques were instrumental in turning NVs into some of the world’s most sensitive magnetometers [5]. The techniques used by de Leon’s and Yao’s teams include growing diamond without spinful isotopes of carbon—useful to avoid a decrease in the NV coherence time due to interaction of the NV spin with undesired nuclear spins. Another technique used by both groups involves enriching diamond layers with nitrogen atoms, which allows better control on NV-center formation.
The exquisite sensitivity of NV centers, however, can be a double-edged sword. The ability to detect nanotesla magnetic fields means that the NV is also sensitive to unwanted fields from adjacent surfaces and from other defect species present within the diamond crystal [6]. These interactions lead to decoherence—a loss in the quantum information stored in the NV’s electron spin states. The two teams of researchers take important steps in understanding the mechanisms by which interactions with the environment cause the NV’s decoherence.
De Leon’s collaboration looked at a particularly insidious problem related to unpaired electrons at the surface of the diamond crystal. The presence of these defects has long been established, but their effects are still poorly understood, partly because the defects are optically inactive and thus difficult to probe directly. The researchers used a microwave control technique called double electron–electron resonance (DEER) to study how the unpaired electrons affect the decoherence of the signal collected from a single NV. Through time-resolved measurements, they obtained two notable results. First, they use the acquired data to derive a model that accurately describes how the decoherence time depends on the depth of the NV center relative to the surface layer. This knowledge will be useful in designing NV scanning-probe sensors, whose performance will depend on a trade-off between signal amplitude and decoherence: small depths increase the proximity to the probed sample and thus the signal amplitude but also speed up the decoherence and thus reduce the time over which the signal can be measured. Second, de Leon and her colleagues show that, counter to the assumptions of prevailing models, the surface unpaired electrons are mobile. The authors suggest that, with proper material engineering, these defects could be stabilized and controlled such that they could be used in advanced sensors—where the unpaired electrons act as “secondary probes” that “report” to the main NV center, thereby boosting its sensitivity.
Using similar methods that combine DEER and microwave techniques, Yao’s collaboration instead explored the interaction of an NV center with an engineered 2D layer of nitrogen impurities, known as P1 centers. This theoretical and experimental study lays the foundation for quantum simulation and sensing platforms based on a combination of NV and P1 centers. Specifically, the researchers investigate two platforms in which different types of interactions dominate the scene. The first requires the mutual interactions between NV centers to dwarf those between NV centers and any other defects or decoherence sources. The researchers show that they can access this regime when the P1 centers are sparse. With two different microwave-pulse sequences, they could prepare the NV centers so as to selectively turn off the NV centers coupling to P1 centers and to other NV centers. The results show that decoupling an NV from the P1 centers only halved the decoherence, while decoupling it also from other NVs led to an additional sixfold reduction in decoherence. This difference clearly demonstrated that, in this regime, the NV–NV dipolar interaction dominates the NV–P1 interaction. The second platform, based on dense P1 centers, could be particularly interesting for quantum simulation approaches involving multiple species of spins, some of which may not be optically accessible. The team showed that it was indeed possible to transfer qubit states from the bright and therefore easily controllable NV centers to the optically dark P1 centers, proving the feasibility of these approaches.
The two studies make strides relevant to a variety of research directions. First, the sheer number of spin defects that can be generated in platforms such as those used by de Leon’s and Yao’s teams makes them much easier to scale-up than platforms based on trapped ions and neutral atoms—offering greater potential for performing complex condensed-matter quantum simulations. Second, similar approaches will allow researchers to explore different regimes of spin physics by controlling the level of interaction (for instance, via the NV depth or density) and the type of interaction (by using different species, such as NV and P1 centers). The approach could be expanded to other material platforms, for example silicon carbide, which, in addition to P1-like (spin-1/2) and NV-like (spin-1) defects, also hosts spin-3/2 species that could reproduce more complex phenomenology. Finally, ideas for enhancing the NV sensing-resolution via a 2D layer of spins—like the unpaired-electron layer studied by de Leon’s team—may lead to ultrasensitive tools for probing the magnetic properties of exotic materials and biological matter.
Correction (4 January 2023): The text was corrected to accurately describe Yao et al.’s decoupling schemes based on microwave pulses. A previous version incorrectly stated that one such scheme decoupled NV centers from other NV centers only, but the actual decoupling was from both NV and P1 centers.
References
- B. L. Dwyer et al., “Probing spin dynamics on diamond surfaces using a single quantum sensor,” PRX Quantum 3, 040328 (2022).
- E. J. Davis et al., “Probing many-body dynamics in a two dimensional dipolar spin ensemble,” Nat. Phys. (to be published) arXiv:2103.12742v3.
- M. W. Doherty et al., “The nitrogen-vacancy colour centre in diamond,” Phys. Rep. 528, 1 (2013).
- F. Casola et al., “Probing condensed matter physics with magnetometry based on nitrogen-vacancy centres in diamond,” Nat. Rev. Mater. 3, 17088 (2018).
- K. Jensen et al., “Magnetometry with nitrogen-vacancy centers in diamond,” High Sensitivity Magnetometers. Smart Sensors, Measurement and Instrumentation, edited by A. Grosz et al. Vol. 19 (Springer, Cham)[Amazon][WorldCat].
- L. V. H. Rodgers et al., “Materials challenges for quantum technologies based on color centers in diamond,” MRS Bull. 46, 623 (2021).