New Nano Capacity
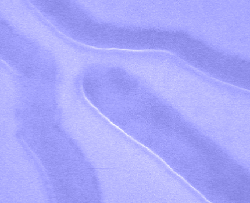
As conventional transistors shrink in size, they lose their ability to amplify signals, and thus their very status as transistors. Now researchers have taken advantage of a proposed quantum effect to construct a Y-shaped, nano electronic circuit that boosts signals spontaneously. The unique device, described in the 25 November print issue of PRL, is one of just a few known designs that might lead to circuits of atomic proportions.
In field-effect transistors, the voltage on a gate electrode controls the flow of electrons through an adjacent wire. A transistor in a circuit is said to amplify because small voltage changes at the gate can lead to larger voltage variations at the output. The gate’s efficiency is determined in part by its capacitance–the amount of charge it can move with a given applied voltage. But as the transistor is scaled down, the gate capacitance decreases and the signal amplification, or gain, is lost. “Everybody’s looking for gain mechanisms in nanoelectronics,” says Lukas Worschech of the University of Würzburg in Germany. “You have to look for new layouts.”
Worschech, Würzburg team leader Alfred Forchel, and their colleagues, chose to explore the electron Y-branch switch (YBS), in which a single stem splits into two branches. Previous work suggested that, at the sizes where an electron’s wave nature becomes important, YBSs would show a “quantum capacitance” [1] which should allow a small YBS to produce gain with a relatively small gate voltage.
Electrons entering the YBS see a road of semiconductor ahead of them forking in two, just like a “Y.” As electrons approach the split, a pair of gate electrodes located just to the left and right of the Y can steer them preferentially down either branch, depending on the voltages applied to the gates. Similar to a classical transistor, a voltage difference between the gates (the input) is amplified as electrons flow differentially through the branches of the Y and create a larger voltage difference between the branches (the output). But for a small enough YBS, the branches of the Y may “self-gate” to some extent, because they can acquire some quantum capacitance, which leads to extra amplification.
To test the idea, Worschech and his colleagues carved a 50-nm-thick Y shape into a layer of gallium arsenide resting on a second layer of aluminum gallium arsenide. As they ramped up the flow of electrons, the gain increased faster than classical physics would predict, reaching a value close to 30. Worschech suspects that quantum capacitance explains the extra gain, because several aspects of the theory fit the data, but he says more experiments are needed to be sure. He says that the intrinsic gating of the YBS may allow researchers to construct circuits from fewer components, because less external gating is needed.
“There is a new transistor principle here,” says Karl Hess of the University of Illinois at Urbana-Champaign, and “there are not many transistor principles around.” This novelty might translate to new applications, he adds. He says that although the device is still 10 times larger than conceivable silicon electronics, it could in principle be brought down to atomic size.
–JR Minkel
JR Minkel is a freelance science writer in New York City.
References
- J.-O. J. Wesström, Phys. Rev. Lett., 82, 2564 (1999)