Electron Speedometer
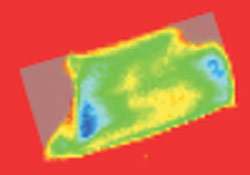
Like traffic cops with radar guns, physicists can now gauge the speed of electrons in a current. In the 27 January PRL, researchers describe a speed-measuring system that uses magnetic resonance and demonstrate a test in which they verified Ohm’s Law by directly observing electrons. The technique could eventually create the first images of the flow of electrons through materials at the micron scale.
The electric current in a material is not the same as the electron velocity but more like the number of cars that pass a specific highway exit per hour. A high car current could mean one lane of fast cars or many lanes of slow traffic. So Elmar Dormann of the University of Karlsruhe in Germany and his colleagues developed a technique to measure electron velocity, separate from current.
Their technique uses magnetic resonance imaging (MRI) technology but is not yet capable of making images. Like all MRI systems, it detects particles by monitoring their spin. In a magnetic field, the spin axis of each electron rotates, or precesses, around the field, just as a tilted, spinning gyroscope or top precesses around the vertical gravitational field. The precession generates radio waves whose frequency depends on the magnetic field strength. Now suppose that field strength varies from place to place inside a sample of material–say, strongest at one end and weakest at the other end, as in all MRI machines. The electrons’ radio wave frequencies will vary with their positions just as the field does.
To measure electron velocity, the team first ran the experiment with no current flowing through a crystal. They applied a short pulse of microwaves to start all the electrons precessing. Although they started out in synch, electrons in different places soon got out of step because of their different local magnetic fields. But before long, a second pulse forced all the spins to jump ahead in their cycles by different amounts, reversing their order, with the slowest-precessing spin in the lead and the fastest behind. After the same period of time, this re-ordering caused the spins to line up again, just where they started, and generate a radio “echo”. It was as though runners on a racetrack suddenly stopped five seconds into the race and then ran backwards for five seconds, putting them all at the start simultaneously. When the team performed the same experiment with a current flowing, many electrons were on the move, exploring a whole range of magnetic field strengths and precession speeds as they moved through the sample. So the second pulse did not re-synchronize all of the spins, and analysis of the radio echo showed how fast they moved.
The spins of protons are relatively easy to detect with MRI, but electron spins randomize more quickly following a pulse. So Dormann and his colleagues used a so-called radical cation salt crystal, a material where it takes microseconds for the electron spins to randomize, rather than nanoseconds, as in ordinary metals. Another major technical problem was that the electron current made its own magnetic field. The team managed to cancel it out by subtracting echoes from two runs with different field arrangements.
As a demonstration, the team showed that electron velocity depends on current in just the way classic theory predicts. But ultimately they hope to image the motion of electrons in a variety of materials. “The work is lovely because the theory just fits so well, and there, at the end of it all, is Ohm’s Law!” says Paul Callaghan of Victoria University in Wellington, New Zealand. Callaghan cautions, however, that the technique may be very difficult to replicate in other materials.
–Kim Krieger
Kim Krieger is a freelance science writer in Norwalk, Connecticut.