The Force of Empty Space
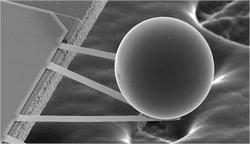
According to quantum mechanics, the vacuum is not empty, but teeming with virtual particles that constantly wink in and out of existence. One strange consequence of this sea of activity is the Casimir effect: Two flat metal surfaces automatically attract one another if they get close enough. The Casimir force is so weak that it has rarely been detected at all, but now a team reports in the 23 November PRL that they have made the most precise measurement ever of the phenomenon. They claim that their technique, using an atomic force microscope, has the capacity to test the strangest aspects of the Casimir effect, ones that have never before been tested.
The simplest explanation of the Casimir effect is that the two metal plates attract because their reflective surfaces exclude virtual photons of wavelengths longer than the separation distance. This reduces the energy density between the plates compared with that outside, and–like external air pressure tending to collapse a slightly evacuated vessel–the Casimir force pulls the plates toward one another. But the most puzzling aspect of the theory is that the force depends on geometry: If the plates are replaced by hemispherical shells, the force is repulsive. Spherical surfaces somehow “enhance” the number of virtual photons. There is no simple or intuitive way to tell which way the force will go before carrying out the complicated calculations.
Since the discovery of the theory by Casimir 50 years ago, there have been only two previous documented detections of the effect. One was in 1958 and had 100% uncertainty, and the second was last year [Phys. Rev. Lett. 78, 5 (1997)], when the theory was verified to within 5%. Umar Mohideen and Anushree Roy, of the University of California at Riverside, claim their new results verify the theory to within 1%.
Mohideen and Roy exploited the exquisite sensitivity of the atomic force microscope (AFM), which can sense forces as small as newtons. In an AFM, the force causes a slight deflection of a microscopic cantilever, which is detected with a laser system. The team affixed an aluminum-plated, 200-µm-diameter sphere to the cantilever and recorded the deflection as it approached a flat, aluminum-plated surface to within 100 nm. They corrected the raw data for several small effects, including the electrostatic force between the surfaces caused by a small excess charge on the sphere. Because the approach was so close, the team also had to correct the theoretical curve to account for the microscopic roughness of the metal surfaces, which they measured using the AFM in a more traditional mode. They applied a further correction to the ideal theory to account for the lack of perfectly reflecting surfaces.
Mohideen says the new method is superior to previous ones because the electrostatic corrections to the data amounted to only a few percent of the size of the Casimir force, whereas in the previous experiment, those corrections were 5 times the Casimir force. But the real importance of the new technique, says Mohideen, is that its precision can be dramatically improved, allowing studies of the weird geometry dependence of the Casimir effect and even its predicted dependence on temperature.
Lawrence Ford, of Tufts University in Medford, MA, says the work is important because any fundamental theory requires verification by more than one research group. But in addition, the latest measurements included separations six times smaller than the experiment last year, so that the researchers could put the corrections to a much more rigorous test. Verifying the theoretical corrections to Casimir’s original theory assures physicists that “[we] really understand what’s going on,” says Ford.