The World of Quantum Chaos
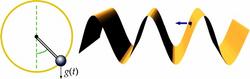
Researchers have studied chaos in classical physics in systems ranging from the motions of the planets to the patterns in weather. Over a long enough time period–millions of years for planetary orbits, a week or two for the weather–the tiniest difference in starting conditions grows to have a huge effect, making chaotic systems erratic and unpredictable. The theory of quantum mechanics, by contrast, seems to preclude this classical type of chaos, allowing only its own distinct varieties. This is a puzzling situation, because the classical world we perceive somehow arises out of the underlying quantum world. In the PRL issues of 11 May and 10 August two teams report using clouds of cesium atoms to observe clear evidence of a quantum chaotic system showing “decoherence,” a phenomenon that is believed to play a major role in the transition from quantum to classical behavior. The experiments provide one glimpse of the strange world where quantum, classical, and chaotic phenomena meet and should allow for more exploration into that poorly understood realm.
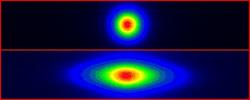
One of the simplest chaotic systems is the kicked rotor. A rigid pendulum rotates on a pivot and is periodically “kicked,” as if gravity were turned on only in brief pulses. Instead of swinging like a pendulum, the rotor moves about erratically, following no regular pattern. If two kicked rotors are set in motion from slightly different starting points, they quickly evolve to very different angles and momenta.
A quantum-scale analog of the rotor is a cold cesium atom in a pulsed standing wave of laser light. During each brief moment that the light is on, the horizontal force on the atom depends on its horizontal position between adjacent wave crests in exactly the same way that the force on the classical rotor depends on its angular position. A hallmark of this quantum chaotic system is “dynamical localization,” an effect first seen in a similar system of sodium atoms a few years ago: The series of kicks spreads out the momentum distribution of the atoms more slowly than classical physics would predict. Roughly speaking, the average speed of the atoms grows more slowly than in a classical system because many of the atoms’ classical chaotic paths interfere destructively. Just as light waves going through a double slit create dark bands where the waves cancel out, the wavelike atoms have zero probability of appearing on many of the classical paths where they would acquire high momentum.
To learn about the relationships between classical and quantum chaos, the two experimental groups (from the University of Texas at Austin and the University of Auckland in New Zealand) set out to observe decoherence–the loss of quantum characteristics–in their chaotic quantum systems. By adjusting the light fields in the experiments, the groups could increase the rate at which their atoms spontaneously emitted photons. Some of the quantum nature of the parent atom “leaks away” into the environment with these spontaneously emitted photons. The waves for different paths no longer interfere so completely.
Both teams observed that greater rates of spontaneous emission caused the momentum distributions of the kicked atoms to spread faster, showing less dynamical localization and behaving more like classical particles. However, the experimental results differed in several important aspects: The Auckland group reported exponential lineshapes (usually considered a characteristic of localization) even in the presence of spontaneous emission. In contrast, the Austin researchers saw lineshapes that bulged out and became non-exponential when they applied spontaneous emission or external noise. This effect is not yet explained by theory.
“The experiments are exploring an interesting regime that is neither fully quantum nor fully classical,” says Salman Habib of Los Alamos National Laboratory. He believes they will lead to better understanding of the connections between the classical and quantum worlds, particularly in the difficult circumstances of chaotic systems. But he adds that, although the experiments are well done, “The theoretical interpretation of the results needs a lot more work.”
–Graham P. Collins
Graham Collins is a freelance science writer.