Self-forming Rings and Cages
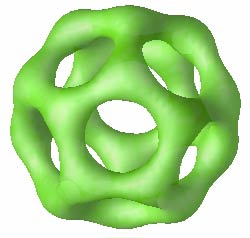
Add certain long-chain molecules to water, and they will condense into spheres, complex rings, and figure-eights. Researchers have not understood exactly how and why the more complex of these shapes form, but a new computer simulation, described in the 4 April Physical Review Letters, shows that the unusual shapes have a separate formation pathway from simpler structures. It also predicts a new cage structure that hasn’t been seen in the lab. The results may help experimentalists better control these structures for potential uses as drug delivery vehicles and as templates for nanofabrication.
In biology, large structures like DNA and proteins spontaneously build up out of smaller building blocks. Chemists can mimic this self-assembly to make other useful structures with synthetic compounds. One example is the hybrid molecules called copolymers, made of two different polymer strands fastened together end-to-end, to make a single, long chain. When placed in solution, one end of the copolymer is attracted to the solvent while the other end is repelled, similar to the oily molecules called lipids that make up biological cell membranes. This property causes copolymers or lipids to group together into spheres or bi-layered sheets, with their solvent-philic heads pointing outside and their solvent-phobic tails hiding inside. These structures are called micelles, and the non-biological kind remain somewhat mysterious. “Although the various shapes of micelles were discovered in experiments, people do not know why and how they are formed,” says Xuehao He of Tianjin University in Tianjin City, China.
To systematically visualize what is going on, He and Friederike Schmid of Bielefeld University in Germany have run computer simulations of the formation of complex copolymer micelles. The researchers calculated the changes in polymer density over 10-nanometer space increments and microsecond time steps. They varied just two parameters: the copolymer concentration and the attraction between the solvent and the solvent-philic head. Experimenters usually control this attraction by adding a salt or a second solvent to the solution.
For each set of parameters, He and Schmid let their simulation run for the equivalent of about a second of real time, which is long enough to form structures of around 300 copolymers with diameters of 300-500 nanometers. The simulation generated complex micelles–rings, multi-holed tori, and never-before-seen cage structures [1] . But the team found that these shapes form only near the “critical” copolymer concentration. At lower concentrations, micelles cannot stay together because there are not enough molecules to overcome their tendency to spread out. Far above this critical concentration, the simulation only made simple micelles, including solid spheres, rods, and solvent-filled shells called vesicles.
The team was able to discern two separate formation pathways. In the first, copolymers collect into tiny droplets, which later merge to form rods, then sheets, and finally vesicles. But this scenario is unlikely to form rings and cages because the ends of rods are not physically attracted to each other. Instead, there is a second pathway, in which droplets grow bigger not by merging but by absorbing more copolymers from solution. Eventually, one of these spheres becomes so big that a cavity opens up inside. Several holes or tears can form in the expanding shell, and the structure settles into a ring or a more complex torus. According to the authors, experimenters may be able to control the final shape by starting the process with already-formed micelle “seeds,” or by working with mixtures of different copolymer types.
The simulation “provide[s] some guidance as to how to explore parameter space to find unusual shapes, and that could be very useful,” says Tim Lodge of the University of Minnesota. Jeroen Cornelissen of Radboud University in the Netherlands says that he and others are exploring how new shapes may improve functionality. For example, a micelle loaded with a drug may move more easily through the body towards its target when it has a particular shape.
–Michael Schirber
Michael Schirber is a Corresponding Editor for Physics Magazine based in Lyon, France.
References
- Only one previous simulation has shown similarly complex structures, but it used a narrower range of parameters and didn’t start with a homogeneous solution: J. G. E. M. Fraaije and G. J. A. Sevink, “Model for Pattern Formation in Polymer Surfactant Nanodroplets,” Macromolecules 36, 7891 (2003)