Electrons Favor One Direction over Others
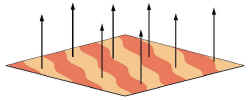
Water waves traveling from north to south across a lake should act just like those going from east to west. Electrons moving through an isotropic sample ought to behave the same way, so the physicists who first saw differences in the electrical resistance of such a sample, depending on the direction they measured, assumed it was a mistake. Now they have confirmed those data with results published in the 11 January PRL. The curious behavior occurred with electrons confined to a plane at very low temperatures in the presence of a magnetic field, and researchers hope it will lead to a deeper understanding of electron interactions.
The combination of confinement, magnetic field, and low temperature leads to a set of discrete, widely-spaced electron energy levels known as Landau levels. As the field is turned up, the spacing between levels increases, so that a constant number of electrons is divided among fewer levels, with all electrons eventually inhabiting the lowest level when a very strong field is applied. During this process of increasing the magnetic field, the highest energy electrons drop down from one Landau level to the next, and the sample’s resistance goes through complicated patterns, including many peaks and zeroes. After two Nobel prizes in the field [see Focus story from 1998], physicists can explain the basic features of the resistance data with two different types of models: At low magnetic fields, when electrons populate higher Landau levels, physicists imagine that the electrons act independently and do not interact (the “integer quantum Hall effect”). But at very high magnetic fields, when electrons occupy only the lowest level, the observations are explained by a single quantum state that includes strong interactions among electrons (the “fractional quantum Hall effect”). There is also an overlap regime, where both types of effects occur.
When Jim Eisenstein, now of the California Institute of Technology, and his colleagues observed strange direction-dependent resistance in quantum Hall data more than a decade ago, “we thought it was some kind of garbage,” Eisenstein recalls. Now he has revisited those experiments with new collaborators and extremely clean samples. The team used a sandwich of two semiconducting crystals that confine electrons between their pristine surfaces, and they were careful to rotate the crystals during growth to avoid favoring any direction in the structure. But they still found a dramatic anisotropy: Comparing the resistance of the sample in two perpendicular directions (both perpendicular to the magnetic field), they found the value in one direction was up to one hundred times its value in the other direction. This strange effect occurred only at magnetic fields where the third Landau level was occupied; it disappeared at stronger fields.
The results remain unexplained, although theorists are favoring a scenario based on a collective state of strongly interacting electrons–akin to that in the fractional quantum Hall effect–but one where a single direction in space is preferred. How that direction gets chosen is “the big bugaboo, the thing that nobody has a clue about,” says Eisenstein, although he assumes it is some very weak effect, just as a knitting needle balanced on its tip will fall in the direction favored by the slightest vibration or material imperfection. The most popular description of the anisotropic state is a type of “charge density wave” where the interacting electrons pile up into “stripes”–alternating regions of higher and lower electron population–rather than remaining evenly distributed throughout the material.
“It’s very important,” says Eduardo Fradkin of the University of Illinois in Urbana, “because it tells you that there are other electron phases,” besides those of the integer and fractional quantum Hall effects. He says the new results demonstrate that the quantum Hall system is an “ideal laboratory to study electron correlation.”