Fickle Magnet Switches Poles Twice
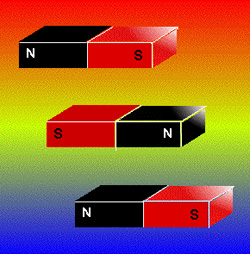
Condensed matter physicists can precisely control many aspects of material properties, but magnetic characteristics are difficult to engineer. Now a team of physicists in Japan has controlled the magnetic properties of a material in a new way. In the 8 February PRL the team reports their design and preparation of a material that flips the orientation of its magnetic field twice as its temperature is increased. They successfully predicted this property based on a simple theoretical model.
Iron is good for making permanent magnets because it’s ferromagnetic: The tiny magnetic field created by each atom tends to line up with its neighbors in the same direction. Antiferromagnetic materials, in contrast, make bad magnets because atomic neighbors tend to align antiparallel to one another, creating a zero net magnetic field. In a third class of materials called “ferrimagnets” the cancellation of neighboring atoms is incomplete, so a net magnetic field remains. But the direction of this “residual magnetization” can switch as a ferrimagnet is heated up because the relative strengths of the two canceling components can change with temperature.
The Japanese team, led by Kazuhito Hashimoto of the University of Tokyo, created a material that flips the orientation of its field at 33 and 53 K before losing all magnetism at 61 K , where thermal motion overwhelms the atoms’ magnetic interactions. They used a “Prussian-blue analog,” a material with a cubic crystal structure. In this structure they introduced atoms of iron, chromium, nickel, and manganese, creating interpenetrating sublattices in the structure that independently behave as ferromagnets which partially cancel one another. The total magnetization of the crystal at any temperature is the sum of contributions from the sublattices, each of which has magnetic strengths with different temperature dependences. Since the sublattice fields cancel to some extent, the combined behavior leads to a type of ferrimagnet that switches orientation at two temperatures.
Hashimoto and his colleagues were able to predict the properties of the material using molecular field theory. Normally this theory requires extensive computations of the interactions between each atom and many of its neighbors, but the Japanese team was able to consider only “nearest neighbors” because of the long distances between the metal atoms. “Consequently the molecular field theory becomes very simple,” says Hashimoto. Using this technique, he says, “we can easily design the magnetic properties of a material.”
George Sawatzky of the University of Groningen in the Netherlands says that the work shows how well certain magnetic systems are now understood, although molecular field theory is a somewhat crude approach. “This is one of the first systems to which you can apply a very simple molecular field theory and make predictions, and then confirm these predictions. It is rather surprising that it works as well as it does.”
–Alexander Hellemans
Alexander Hellemans is a freelance science writer in Naples, Italy, and a contributor to the upcoming book 30-Second Quantum Theory (Icon Books, June 2014).